Case Study 25. Parallel Paradigms: Umbrella Hypotheses and Aquatic Apes
- First Online: 26 October 2016

Cite this chapter
- John H. Langdon 2
2560 Accesses
An umbrella hypothesis is an evolutionary scenario built around a premise that offers answers for a wide range of adaptive problems. Umbrella hypotheses have a deceptive appearance of parsimony because the explanations they offer for independent characters are hypotheses that are no better tested or supported with evidence than before. Instead, another layer of untestable hypothesis has been added. The example examined in this chapter is the Aquatic Ape Hypothesis, which proposes that important human characteristics were originally adaptations to an ecological niche in or near the water. Unlike many conventional umbrella hypotheses, this one emerged within a separate paradigm that has not yet been reconciled to mainstream paleoanthropology.
This is a preview of subscription content, log in via an institution to check access.
Access this chapter
Subscribe and save.
- Get 10 units per month
- Download Article/Chapter or eBook
- 1 Unit = 1 Article or 1 Chapter
- Cancel anytime
- Available as PDF
- Read on any device
- Instant download
- Own it forever
- Available as EPUB and PDF
- Compact, lightweight edition
- Dispatched in 3 to 5 business days
- Free shipping worldwide - see info
- Durable hardcover edition
Tax calculation will be finalised at checkout
Purchases are for personal use only
Institutional subscriptions
Additional Reading
Kuliukas AV, Morgan E (2011) Aquatic scenarios in the thinking on human evolution: what they and how do they compare? In: Vaneechoutte M et al (eds) Was man more aquatic in the past? Fifty years after Alister Hardy: waterside hypotheses of human evolution. Bentham eBooks, Oak Park, pp 106–119
Google Scholar
Langdon JH (1997) Monolithic hypotheses and parsimony in human evolution: a critique of the aquatic ape hypothesis. J Hum Evol 33:479–494
Article CAS PubMed Google Scholar
Download references
Author information
Authors and affiliations.
University of Indianapolis, Indianapolis, IN, USA
John H. Langdon
You can also search for this author in PubMed Google Scholar
Rights and permissions
Reprints and permissions
Copyright information
© 2016 Springer International Publishing Switzerland
About this chapter
Langdon, J.H. (2016). Case Study 25. Parallel Paradigms: Umbrella Hypotheses and Aquatic Apes. In: The Science of Human Evolution. Springer, Cham. https://doi.org/10.1007/978-3-319-41585-7_25
Download citation
DOI : https://doi.org/10.1007/978-3-319-41585-7_25
Published : 26 October 2016
Publisher Name : Springer, Cham
Print ISBN : 978-3-319-41584-0
Online ISBN : 978-3-319-41585-7
eBook Packages : Biomedical and Life Sciences Biomedical and Life Sciences (R0)
Share this chapter
Anyone you share the following link with will be able to read this content:
Sorry, a shareable link is not currently available for this article.
Provided by the Springer Nature SharedIt content-sharing initiative
- Publish with us
Policies and ethics
- Find a journal
- Track your research
A New Aquatic Ape Theory
/https://tf-cmsv2-smithsonianmag-media.s3.amazonaws.com/accounts/headshot/science-erin-wyman-240.jpg)
Erin Wayman
/https://tf-cmsv2-smithsonianmag-media.s3.amazonaws.com/filer/20120416015020GorillaSmall.jpg)
The aquatic ape theory, now largely dismissed, tries to explain the origins of many of humankind’s unique traits. Popularized in the 1970s and 1980s by writer Elaine Morgan , the theory suggests that early hominids lived in water at least part of the time. This aquatic lifestyle supposedly accounts for our hairless bodies, which made us more streamlined for swimming and diving; our upright, two-legged walking, which made wading easier; and our layers of subcutaneous fat, which made us better insulated in water (think whale blubber). The theory even links an aquatic existence to the evolution of human speech .
The hypothesis was met with so much criticism that it’s not even mentioned in human evolution textbooks. But that doesn’t mean aquatic habitats didn’t play some kind of role in our ancestors’ lives.
In 2009, Richard Wrangham of Harvard University and colleagues suggested in the American Journal of Physical Anthropology ( PDF) that shallow aquatic habitats allowed hominids to thrive in savannas, enabling our ancestors to move from tropical forests to open grasslands.
About 2.5 million to 1.4 million years ago, when the genus Homo emerged, Africa became drier. During certain seasons, already dry savannas became even more arid, making it difficult for hominids to find adequate food. But Wrangham’s team argues that even in this inhospitable environment there were oases: wetlands and lake shores. In these aquatic habitats, water lilies, cattails, herbs and other plants would have had edible, nutritious underground parts—roots and tubers—that would have been available year-round. These “fallback” foods would have gotten hominids through the lean times.
The researchers based their arguments on modern primate behavior. For example, baboons in Botswana’s Okavango Delta , which floods every summer, start eating a lot of water lily roots when fruit becomes scarce. And hunter-gatherers in parts of Africa and Australia also eat a lot of roots and tubers from aquatic plants.
The fossil record also hints at the importance of aquatic environments. Wrangham and his team looked at nearly 20 hominid fossil sites in East and South Africa. In East Africa, the geologic and fossil evidence suggests that hominids were living in areas with lakes or flooded grasslands. South African sites tended to be drier but were still located near streams.
The researchers say foraging in these environments may have led to habitual upright walking. Today, chimpanzees and gorillas occasionally venture into shallow bodies of water, and when they do, they wade on two legs. It makes sense. Wading bipedally allows the apes to keep their heads above water. As our earliest ancestors spent longer and longer periods of time wading upright, it became beneficial to evolve specialized anatomy for two-legged walking.
Wrangham and his colleagues acknowledge that their case rests on circumstantial evidence. There’s no direct proof that this is how hominids were living. And the evidence has alternative explanations. For instance, watery habitats allow for better fossil preservation, so finding hominids in watery locales may not be representative of where they actually spent most of their time.
So like most things in human evolution, the debate’s wide open. What role do you think wetlands and lake shores played in our ancestors’ lives?
Get the latest Science stories in your inbox.
/https://tf-cmsv2-smithsonianmag-media.s3.amazonaws.com/accounts/headshot/science-erin-wyman-240.jpg)
Erin Wayman | | READ MORE
Erin Wayman is a science and human evolution blogger for Hominid Hunting. She has M.As in biological anthropology and science writing.
This page has been archived and is no longer updated
Primate Cognition

Cognition is the ability to interpret and connect information that is perceived in the world and to then apply this personal knowledge adaptively to future situations and problems (Cheney & Seyfarth, 1990). Importantly, this allows the animal to improve their fitness . All animals have to negotiate their environment to allow them to forage for food, avoid predation and find mates. Primates stand out among other taxa for their flexibility in how they deal with the world around them. They inhabit both complex physical and social worlds, which have each been posited as the major selective pressures driving the advancement of primate brain size and, by extension, their cognitive abilities (Byrne, 2000; Reader et al. , 2011); accordingly we first describe their understanding of their physical environment and then go on to discuss social cognition.
Primate Cognition about the Physical World
Cognitive mapping, memory and future planning, tool-use behavior and causal understanding, social cognition, cooperation and prosocial behaviour.
While cooperation is common, prosocial behaviour is more rare. There is little evidence in apes that individuals change their behaviour to bring a partner food (e.g. Silk et al. , 2005; but see Horner et al. , 2011), although several monkey species do so (e.g. de Waal et al. , 2008; Burkart et al. , 2007; Massen et al. , 2010), even in cases in which being prosocial actually results in inequity towards the provider (Brosnan et al. , 2010). On the other hand, even among chimpanzees, behaviours which help a partner are seen outside of the food context (e.g., helping; Warneken & Tomasello, 2006). One hypothesis is that prosocial behaviours are more common among cooperative breeders , due to the unique costs and benefits related to their interactions with each other (Blaffer-Hrdy, 2009), leading them to more actively share food with partners (Jaeggi et al. , 2010).
Machiavellian and social Intelligence
Social learning.
There is clearly a marked difference, however, between human culture and the behavioural traditions of primates, and what underlies this contrast is currently a topic of much debate. Cumulative culture has been proposed to describe the underlying mechanism that has allowed for the development of our complex technologies. Without the ability for imitation or teaching , researchers argue, primates may not be capable of cumulative learning because the complex information required for such intricate behaviours cannot be transmitted faithfully (Dean et al ., 2014; Tomasello, 1999). Experimental studies with humans, however, reveal that teaching and imitation are not prerequisite for cumulative culture (Caldwell & Millen, 2009; Caldwell et al ., 2012). Given this, along with the mounting evidence that primates are able to copy even complex tool-use behaviours from observing others (Price et al. , 2009), what can explain the relative paucity of the cultural worlds of our closest-living relatives, especially for the chimpanzee? It may well be that the very ability of chimpanzees to copy others leads to their limited ability to build on the knowledge of previous generations. After learning a specific method from observations of others, chimpanzees appear to become entrenched and unable to transition to a new behaviour, even if the introduced method is more efficient (Hopper et al. , 2011). Although seemingly a limitation of their learning capacities, such as conformity and conservatism may enable chimpanzees to maintain strong social bonds and potentially avoid risks in the wild. Thus, the key ability here is not just in copying others, but determining when to do so (Rendell et al. , 2011).
Conclusions
cognitive mapping : "The representation embodied in a cognitive map is typically assumed to encode distances and directions and to enable mental operations of them" (Shettleworth, 1998, p.296) allowing animals to calculate the optimum routes from point A to point B.
conservatism : Mastery of a skill inhibits further exploration to new alternative options or behavioural strategies which may be more efficient
cooperation : Two or more individuals increase their direct fitness more by working together than individually (Brosnan, Salwiczek & Bshary, 2010).
cooperative breeders : Social system in which young are cared for by both parents and, often, their mature offspring.
cumulative culture : The process by which generations build upon the knowledge of previous ones creating increasingly complex artefacts and technologies through the accumulation of design — the so-called "ratchet effect" (Tomasello, 1999).
deception : The provisioning of false information which affects another individual's behaviour in favour of the deceiver. While this may occur without intention on the part of the deceiver, resulting in functional, or tactical, deception, individuals may also do so intentionally.
fission-fusion : In which a group of individuals breaks ups and reforms over the course of a day or week meaning that each party size represents a subset of total group size.
fitness : The ability to survive and successfully reproduce (e.g., evolutionary fitness)
home range : The territory which a primate, or group of primates, occupies. Note, primates move about their territory but may only travel within small subsections each day. Furthermore, the degree to which a primates' home range overlaps with conspecifics and different primate species is affected not just by the specific primate but also by environmental and space pressures (e.g., food availability and deforestation).
imitation : Copying the exact behaviours demonstrated by another. Compare with ‘emulation' in which an individual merely replicates the goal or end-state of a novel behaviour performed by others.
inequity : A situation in which unequal rewards are received. The behavioural response studied is ‘inequity aversion' vis-à-vis a sense of unfairness (see Brosnan, 2006, for further discussion).
prosocial behaviour : Behaviour which results in a benefit to another individual.
teaching : "An individual actor A can be said to teach if it modifies its behaviour only in the presence of a naive observer, B, at some cost or at least without obtaining an immediate benefit for itself. A's behaviour thereby encourages or punishes B's behaviour, or provides B with experience, or sets an example for B. As a result, B acquires knowledge or learns a skills earlier in life or more rapidly or efficiently than it might otherwise do, or that it would not learn at all" (Caro & Hauser, 1992, p. 153).
theory of mind : The attribution of mental states such as beliefs, thoughts, desires and intentions to oneself and others. See Heyes (1998) for a detailed discussion about primate understanding of theory of mind.
traditions : "enduring behaviour patterns shared among members of a group that depend to a measurable degree on social contributions to individual learning, resulting in shared practices among members of a group" (Fragaszy & Perry, 2003, p.3)
working memory : The short-term retention of information which may sometimes incorporate elements of information processing.
References and Recommended Reading
Recommended reading:.
Byrne, R. W. The Thinking Ape: The Evolutionary Origins of Intelligence . Oxford, UK: Oxford University Press (1995).
de Waal, F. B. M. & Ferrari, P. F. The Primate Mind: Built to Connect with Other Minds. Cambridge, MA: Harvard University Press (2012).
Maestripieri, D. Primate Psychology . Cambridge, MA: Harvard University Press (2005).
Tomasello, M. & Call, J. Primate Cognition . Oxford, UK: Oxford University Press (1997).
References:
Asensio, N., Brockelman, W. et al. Gibbon travel paths are goal oriented. Animal Cognition 14 , 395-405 (2011).
Ban, S., Boesch, C. & Janmaat, K. L. Taï chimpanzees anticipate revisiting high-valued fruit trees from further distances. Animal Cognition (EPUB 2014) doi:10.1007/s10071-014-0771-y.
Bates, L. A. & Byrne, R. W. Sex differences in the movement of free-ranging chimpanzees ( Pan troglodytes schweinfurthii ). Behavioral Ecology & Sociobiology 64 , 247-255 (2009).
Blaffer-Hrdy, S. Mothers and Others: The Evolutionary Origins of Mutual Understanding . Cambridge, MA: Belknap Press of Harvard University Press (2009).
Boesch, C. Cooperative hunting in wild chimpanzees. Animal Behavior 48 , 653-667 (1994).
Boysen, S. T. & Berntson, G. G. Numerical Competence in a Chimpanzee ( Pan troglodytes ). Journal of Comparative Psychology 103 , 23-31 (1989).
Brosnan, S. F. Nonhuman species' reactions to inequity and their implications for fairness. Social Justice Research 19 , 153-185 (2006).
Brosnan, S. F. A hypothesis of the co-evolution of inequity and cooperation. Frontiers in Decision Neuroscience 5 , 43 (2011).
Brosnan, S. F. & Bshary, R. Cooperation and deception: from evolution to mechanisms. Philosophical Transactions of the Royal Society London B 365 , 2593-2598 (2010).
Brosnan, S. F. & Hopper, L. M. Cooperation, behavioral diversity and inequity responses. In M. Banaji & S. Gelman (Eds.) Navigating the Social World: What Infants, Children, and Other Species Can Teach Us. (pp. 371-376). New York: Oxford University Press (2013).
Brosnan, S. F., Houser, D. et al. Competing demands of prosociality and equity in monkeys. Evolution & Human Behavior 31 , 279-288 (2010).
Brosnan, S. F., Parrish, A. R. et al. Responses to the assurance game in monkeys, apes, and humans using equivalent procedures. Proceedings of the National Academy of Sciences 108 , 3442-3447 (2011).
Burkart, J. M., Fehr, E. et al. Other-regarding preferences in a non-human primate: Common marmosets provision food altruistically. Proceedings of the National Academy of Sciences 104 , 19762-19766 (2007).
Byrne, R. W. Evolution of primate cognition. Cognitive Science 24 , 543-570 (2000).
Byrne, R. W. & Whiten, A. Machiavellian Intelligence: Social Expertise and the Evolution of Intellect in Monkeys, Apes, and Humans . Oxford, UK: Clarendon Press (1988).
Caldwell, C. A. & Millen, A. E. Social learning mechanisms and cumulative cultural evolution: Is imitation necessary? Psychological Science 20 , 1478-1483 (2009).
Caldwell, C. A., Schillinger, K., Evans, C. L. & Hopper, L. M. End state copying by humans (Homo sapiens): Implications for a comparative perspective on cumulative culture. Journal of Comparative Psychology 126 , 161-169 (2012).
Caro, T. M. & Hauser, M. D. Is there teaching in nonhuman animals? The Quarterly Review of Biology 67 , 151-174 (1992).
Cheney, D. L. & Seyfarth, R. M. How Monkeys See the World: Inside the Mind of Another Species . Chicago, IL: University of Chicago Press (1990).
Cook, P. & Wilson, M. Do young chimpanzees have extraordinary working memory? Psychological Bulletin & Review 17 , 599-600 (2010).
Dean, L. G., Vale, G. L. et al . Human cumulative culture: a comparative perspective. Biological Reviews 89 , 284-301 (2014).
de Waal, F. B. M. & Davis, J. M. Capuchin cognitive ecology: Cooperation based on projected returns. Neuropsychologia 1492 , 1-8 (2002).
de Waal, F. B. M., Leimgruber, K.& Greenberg, A. Giving is self-rewarding for monkeys. Proceedings of the National Academy of Sciences 105 , 13685-13689 (2008).
Gallup, G. G., Jr. Chimpanzees: Self-recognition. Science 167 , 86-87 (1970).
Gomes, C. M. & Boesch, C. Wild chimpanzees exchange meat for sex on a long-term basis. PLoS ONE 4 , e5116 (2009).
Gomes, C. M., Mundry, R. & Boesch, C. Long-term reciprocation of grooming in wild West African chimpanzees. Proceedings of the Royal Society of London B . 276 , 609-706 (2008).
Hare, B., Addessi, E. et al. Do capuchin monkeys, Cebus apella , know what conspecifics do and do not see? Animal Behaviour 65 , 131-142 (2003).
Hare, B., Call, J. & Tomasello, M. Do chimpanzees know what conspecifics know? Animal Behaviour 61 , 139-151 (2001).
Heyes, C. M. Theory of mind in nonhuman primates. Behavioral and Brain Sciences 21 , 101-148 (1998).
Hopper, L. M. Deferred imitation in children and apes: Children imitate after a delay, but can apes ape in a similar fashion? The Psychologist 23 , 2-5 (2010).
Hopper, L.M., Schapiro, S. J. et al. Chimpanzees' socially maintained food preferences indicate both conservatism and conformity. Animal Behaviour 81 , 1195-1202 (2011).
Horner, V., Carter, J.D., Suchak, M., & de Waal, F.B.M. Spontaneous prosocial choice by chimpanzees. Proceedings of the National Academy of Sciences 108 , 13847-13851 (2011).
Horner, V. & Whiten, A. Learning from others' mistakes? Limits on understanding a trap-tube task by young chimpanzees ( Pan troglodytes ) and children ( Homo sapiens ). Journal of Comparative Psychology 121 , 12-21(2007).
Inoue, S. & Matsuzawa, T. Working memory of numerals in chimpanzees. Current Biology 17 , R1004-R1005 (2007).
Jaeggi, A. V., Burkart, J. M.& Van Schaik, C. P. On the psychology of cooperation in humans and other primates: combining the natural history and experimental evidence of prosociality. Philosophical Transactions of the Royal Society of London B , 365 , 2723-2735 (2010).
Janmaat, K. R. L., Byrne, R. W., & Zuberbühler, K. Primates take weather into account when searching for fruits. Current Biology 16 ,1232-1237 (2006).
Janson, C. H. Experimental evidence for spatial memory in foraging wild capuchin monkeys, Cebusapella . Animal Behaviour 55 , 1229-1243 (1998).
Massen, J. J. M., van den Berg, L. M. et al. Generous leaders and selfish underdogs: Pro-sociality in despotic macaques. PLoS ONE 5 , e9734 (2010).
Melis, A. P., Hare, B. & Tomasello, M. Do chimpanzees reciprocate received favours? Animal Behavior 76 , 951-962 (2008). Melis, A. P., Warneken, F. et al. Chimpanzees help conspecifics obtain food and non-food items. Proceedings of the Royal Society B 278 , 1405-1413 (2011).
Menzel, C. R. Unprompted recall and reported of hidden objects by a chimpanzee ( Pan troglodytes ) after extended delays. Journal of Comparative Psychology 113 , 426-434 (1999).
Mulcahy, N. J. & Call, J. Apes save tools for future use. Science 312 , 1038-1040 (2006a).
Mulcahy, N. J. & Call, J. How great apes perform on a modified trap-tube task. Animal Cognition 9 , 193-199 (2006b).
Olthof, A., Iden, C. M. & Roberts, W. A. Judgments of ordinality and summation of number symbols by squirrel monkeys ( Saimiri sciureus ). Journal of Experimental Psychology 23 , 325-339 (1997).
Ottoni, E. B. & Mannu, M. Semifree-ranging Tufted capuchins ( Cebus apella ) spontaneously use tools to crack open nuts. International Journal of Primatology , 22 , 347-358(2001).
Parr, L. A., Winslow, J. T. et al. Recognizing facial cues: individual discrimination by chimpanzees ( Pan troglodytes ) and rhesus monkeys ( Macaca mulatta ). Journal of Comparative Psychology 114 , 47-60 (2000).
Pouydebat, E., Gorce, P. et al. Substrate optimization in nut cracking by capuchin monkeys ( Cebus apella ). American Journal of Primatology 68 , 1017-1024 (2006).
Price, E. E., Lambeth, S. P. et al. A potent effect of observational learning on chimpanzee tool construction. Proceedings of the Royal Society B 276 , 3377-3383 (2009).
Reader, S. M., Hager, Y. & Laland, K. N. The evolution of primate general and cultural intelligence. Philosophical Transactions of the Royal Society B 366 , 1017-1027 (2011).
Rendell, L., Fogarty, L. et al. Cognitive culture: theoretical and empirical insights into social learning strategies. Trends in Cognitive Sciences 15 , 68-76 (2011).
Sands, S. F. & Wright, A. A. Primate memory: retention of serial list items by a rhesus monkey. Science 209 , 938-940 (1980).
Santorelli, C. J., Schaffner, C. M. et al. Traditions in Spider Monkeys Are Biased towards the Social Domain. PLoS ONE 6 , e16863 (2011).
Schmitt, V. & Fischer, J. Representational format determines numerical competence in monkeys. Nature Communication 2 , 257 (2011).
Shettleworth, S. J. Cognition, Evolution and Behaviour . Oxford, UK: Oxford University Press (1998).
Silk, J. B., Brosnan, S. F. et al. Chimpanzees are indifferent to the welfare of unrelated group members. Nature 437 , 1357-1359 (2005).
Tomasello, M. The Cultural Origins of Human Cognition . Cambridge, MA: Harvard University Press (1999).
vanSchaik, C. P. & Burkart, J. M. Social learning and evolution: the cultural intelligence hypothesis. Philosophical Transactions of the Royal Society B 366 , 1008-1016 (2011).
Vedder, A. L. Movement patterns of a group of free-ranging mountain gorillas ( Gorilla gorillaberingei ) and their relation to food availability. American Journal of Primatology 7 , 73-88 (1984).
Visalberghi, E., Addessi, E. et al. Selection of effective stone tools by wild bearded capuchin monkeys ( Cebus libidinosus ). Current Biology 19 , 213-217 (2008).
Visalberghi, E., & Tomasello, M. Primate causal understanding in the physical and psychological domains. Behavioural Processes 42 , 189-203 (1998).
Warneken, F. & Tomasello, M. Altruistic helping in human infants and young chimpanzees. Science 311 , 1301-1303 (2006).
Wheeler, B. Monkeys crying wolf? Tufted capuchin monkeys use anti-predator calls to usurp resources from conspecifics. Proceedings of the Royal Society B 276 , 3013-3018 (2009).
Whiten, A. The scope of culture in chimpanzees, humans and ancestral apes. Philosophical Transactions of the Royal Society B 366 , 997-1007 (2011).
Article History
Flag inappropriate.


Email your Friend

- | Lead Editor: Jessica Rothman

Within this Subject (9)
- Basic (3)
- Intermediate (5)
- Advanced (1)
Other Topic Rooms
- Ecosystem Ecology
- Physiological Ecology
- Population Ecology
- Community Ecology
- Global and Regional Ecology
- Conservation and Restoration
- Animal Behavior
- Teach Ecology
- Earth's Climate: Past, Present, and Future
- Terrestrial Geosystems
- Marine Geosystems
- Scientific Underpinnings
- Paleontology and Primate Evolution
- Human Fossil Record
- The Living Primates

© 2014 Nature Education
- Press Room |
- Terms of Use |
- Privacy Notice |

Visual Browse

Human Evolution @ UCL
- Research Themes
- Collaborative projects & Networks
- Departments

Miocene Fossil Apes: An updated phylogenetic hypothesis
8 March 2022
The living great apes, humans, and their fossil relatives (Hominidae) are among the most intensively studied mammalian groups, but many aspects of their shared evolutionary history remain poorly understood.
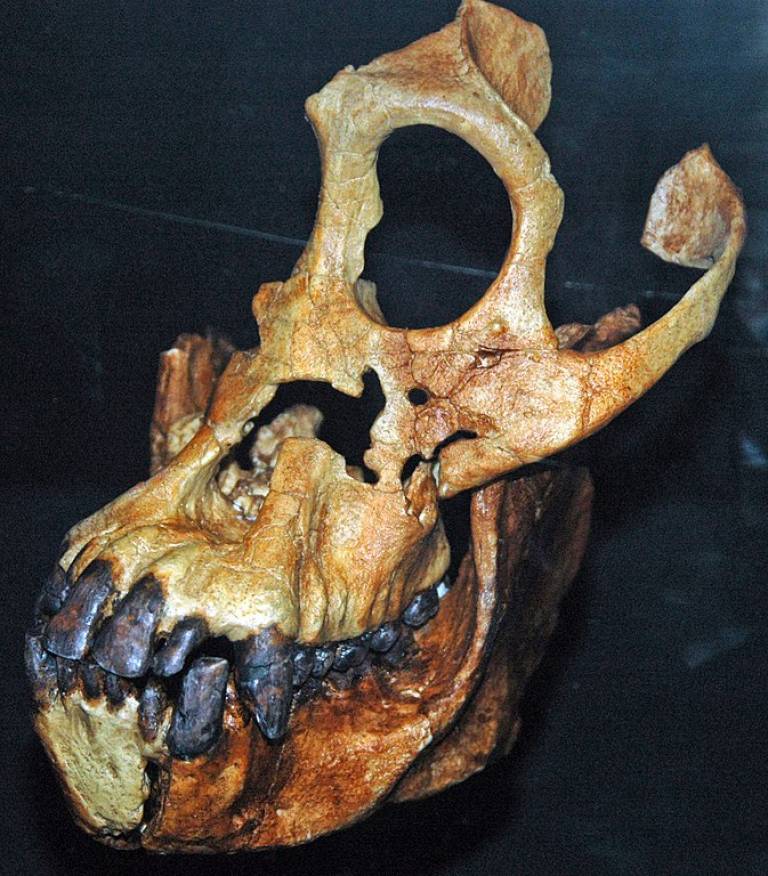
Despite intensive study, many aspects of the evolutionary history of great apes and humans (Hominidae) are not well understood. In particular, the phylogenetic relationships of many fossil taxa remain poorly resolved. This study aims to provide an updated hypothesis of phylogenetic relationships for Middle-Late Miocene fossil apes, focusing on those taxa typically considered to be great apes. The character matrix compiled here samples 274 characters from the skull, dentition, and postcranium. Multiple iterations were performed to examine the effects of ingroup taxon selection, outgroup constraints, treatment of continuous data, character partitions (craniodental, postcranial), and missing data. Parsimony and Bayesian methods were used to infer phylogenetic relationships. Most European hominoids (Hispanopithecus, Rudapithecus, Dryopithecus, Pierolapithecus) are recovered as stem hominids, not more closely related to orangutans or to African apes and humans (Homininae), whereas Ouranopithecus, Graecopithecus, and Nakalipithecus are inferred to be members of the hominine clade. Asian fossil hominoids, with the exception of Lufengpithecus hudienensis, are recovered as part of the orangutan clade (Ponginae). Results suggest that Kenyapithecus and Griphopithecus are possible stem hominids, whereas Equatorius and Nacholapithecus are consistently recovered as stem hominoids. Oreopithecus and Samburupithecus are not recovered as hominids. Results of Bayesian analyses differ from those of parsimony analyses. Craniodental and postcranial character partitions are incongruent in the placement of hylobatids, which is interpreted as evidence that hylobatids and hominids independently evolved adaptations to suspensory positional behaviors. An understanding of phylogenetic relationships is necessary to address many of the questions asked in paleoanthropology. Thus, the updated hypothesis of phylogenetic relationships presented here can be used to gain a better understanding of important morphological transitions that took place during hominid evolution, ancestral morphotypes at key nodes, and the biogeography of the clade.
Phylogenetic analysis of Middle-Late Miocene apes
Kelsey D. Pugh
Related News
HYPOTHESIS AND THEORY article
Apes have culture but may not know that they do.
- 1 Department of Comparative Cognition, Institute of Biology, University of Neuchâtel, Neuchâtel, Switzerland
- 2 School of Psychology and Neuroscience, University of St Andrews, St Andrews, UK
- 3 Cognitive Science Centre, University of Neuchâtel, Neuchâtel, Switzerland
- 4 Anthropological Institute and Museum, University of Zürich, Zürich, Switzerland
There is good evidence that some ape behaviors can be transmitted socially and that this can lead to group-specific traditions. However, many consider animal traditions, including those in great apes, to be fundamentally different from human cultures, largely because of lack of evidence for cumulative processes and normative conformity, but perhaps also because current research on ape culture is usually restricted to behavioral comparisons. Here, we propose to analyze ape culture not only at the surface behavioral level but also at the underlying cognitive level. To this end, we integrate empirical findings in apes with theoretical frameworks developed in developmental psychology regarding the representation of tools and the development of metarepresentational abilities, to characterize the differences between ape and human cultures at the cognitive level. Current data are consistent with the notion of apes possessing mental representations of tools that can be accessed through re-representations: apes may reorganize their knowledge of tools in the form of categories or functional schemes. However, we find no evidence for metarepresentations of cultural knowledge: apes may not understand that they or others hold beliefs about their cultures. The resulting Jourdain Hypothesis, based on Molière’s character, argues that apes express their cultures without knowing that they are cultural beings because of cognitive limitations in their ability to represent knowledge, a determining feature of modern human cultures, allowing representing and modifying the current norms of the group. Differences in metarepresentational processes may thus explain fundamental differences between human and other animals’ cultures, notably limitations in cumulative behavior and normative conformity. Future empirical work should focus on how animals mentally represent their cultural knowledge to conclusively determine the ways by which humans are unique in their cultural behavior.
“Par ma foi! Il y a plus de quarante ans que je dis de la prose sans que j’en susse rien, et je vous suis le plus obligé du monde de m’avoir appris cela.” Mr Jourdain, Le Bourgeois-Gentilhomme, Acte II, scène 4, Molière (1670).
[“By my faith! For more than forty years I have been speaking prose without knowing anything about it, and I am much obliged to you for having taught me that.” Mr Jourdain, The Middle-class Gentleman, Act II, scene 4, Molière (1670) The Gutenberg Project, translation by Philip Dwight Jones].
Ape and Human Cultures: A Difference in Definitions?
Over the last decades, numerous studies have provided evidence for culture-like phenomena in wild animals, especially great apes. Evidence is usually in terms of group-specific behavior patterns ( Whiten et al., 1999 ; Rendell and Whitehead, 2001 ; Perry et al., 2003b ; van Schaik et al., 2003 ), which sometimes involves neighboring groups that live in nearly identical environments and are genetically indistinguishable ( Krützen et al., 2011 ; Gruber et al., 2012a ; Luncz et al., 2012 ). Furthermore, there is good evidence that social learning is the primary force that explains differences between communities in a number of species as opposed to genetic or environmental factors ( Jaeggi et al., 2010 ; Kendal et al., 2010 ; Allen et al., 2013 ; Samuni et al., 2014 ). Building on a long tradition of experimental work in non-primate species ( Warner, 1988 ; Reader and Biro, 2010 ), a promising approach is to use experimental techniques in the wild ( Matsuzawa, 1994 ; Biro et al., 2003 ; Gruber et al., 2009 ), such as seeding a novel behavior to investigate whether it spreads throughout a community ( van de Waal et al., 2010 ).
Although much of this research is still on-going, many scholars now assume that some animal behaviors are ‘cultural’ because they are socially transmitted across generations, thus fulfilling the widely accepted definition of animal traditions as “a distinctive behavior pattern shared by two or more individuals in a social unit, which persists over time and that new practitioners acquire in part through socially aided learning” ( Fragaszy and Perry, 2003 , p. xiii). This definition of traditions would be sufficient to define culture for most biologists, but culture can also be fine-tuned as “the possession of multiple traditions, spanning different domains of behavior” ( Whiten and van Schaik, 2007 , p. 605). However, two sources of skepticism remain. First, an equally influential school of thought argues that ape ‘cultures’ result from convergent rather than homologous processes ( Galef, 2009 ; Tomasello, 2009 ), mainly because of differences in the underlying social learning mechanisms found in humans and other great apes. As a result, apes may be incapable of producing cumulative cultural evolution. Second, for some authors culture is more than a conglomerate of socially acquired behaviors, and should therefore rather be defined as an integrated set of norms that its owners stand for and defend ( Hill, 2009 ; Perry, 2009 ). Whether or not these defining aspects of human culture are also present in animals is currently unknown, which may explain why results from primatology so far have been seen as largely irrelevant by many in the social sciences ( Hill, 2009 ; Perry, 2009 ).
In the following, we review what are currently considered the two major differences between ape and human culture – cumulative culture and normativity – and argue that differences in metarepresentational processes, the cognitive ability to generate representations of representations, underlie these ape-human differences, offering a general explanatory framework. While we acknowledge that it is possible to adopt lower-level explanations to analyze animal behavior, including that of great apes ( Heyes, 1998 ; Shettleworth, 2010 ), we believe that there is compelling evidence that human culture originates from primate roots. Our goal in this article is to distinguish where exactly human and great ape cultures differ to precisely identify what evolved uniquely in the human lineage to generate modern human cultures.
Imitation, Teaching and Cumulative Culture
The first line of argument for a discrepancy between human and animal culture concerns the nature of the underlying mechanisms of social learning. Only human culture, it is argued, results from imitation and teaching, while animal cultures are produced by ‘lower-level’ social learning, such as stimulus enhancement or emulation ( Tomasello, 1990 , 2009 ; Galef, 1992 , 2009 ; Zuberbühler et al., 1996 ; but see Whiten et al., 2009 ). This is supported by the fact that there is generally no good evidence for teaching in non-human primates, in stark contrast to the habitual natural pedagogy found across human societies ( Csibra and Gergely, 2009 ; but see Thornton and Raihani, 2010 for evidence of teaching in non-primate species). The role of imitation in animal culture, however, is more complex. Although chimpanzees can imitate ( Whiten et al., 2007 ; Hobaiter and Byrne, 2010 ), it is not clear whether imitation plays any role in the transmission of behaviors ( Whiten et al., 2009 ) or in maintaining traditions in nature ( Claidière and Sperber, 2010 ).
Similar arguments have been made in relation to ‘cumulative culture,’ which emerges from individuals’ abilities to ‘ratchet’ existing culturally transmitted achievements, that is to “…add an existing technique used in a different context, or an entirely novel technique, to an existing technique, and integrate them functionally” ( Pradhan et al., 2012 , p. 181). Human culture is profoundly more cumulative than anything ever documented in animals, including apes. Composite tools, which are “made of at least two different material elements that are kept together so as to function as one tool” ( Boesch, 2013 , p. 31), are completely lacking in wild chimpanzees although they show evidence for basic cumulative phenomena ( Matsuzawa, 1991 ; Sanz and Morgan, 2007 ; Boesch et al., 2009 ), as do captive chimpanzees ( Yamamoto et al., 2013 ), captive orang-utans ( Lehner et al., 2011 ) and New Caledonian crows ( Hunt and Gray, 2003 ). In sum, more work is needed to confirm the cumulativeness of animal behavior.
Some argue that humans have cumulative culture because only we have both teaching and imitation ( Galef, 2009 ; Hill, 2009 ; Dean et al., 2014 ) but human-animal differences in social learning mechanisms may not be sufficient to explain the emergence of cumulative culture ( Yamamoto et al., 2013 ). Many components of human culture are causally opaque, that is, they cannot be understood or developed by a naïve individual without specific instructions or explanations by a knowledgeable individual. The development of a natural pedagogy to transmit this knowledge may thus have acted as the main force of cumulativeness ( Csibra and Gergely, 2011 ; Pradhan et al., 2012 ). Animal cultures, in contrast, are argued to be causally transparent and to not require instructions to be acquired ( Csibra and Gergely, 2011 ).
Cognitively, intentional teaching and especially pedagogy appear demanding, in that multiple representations must be stored, manipulated, and compared simultaneously ( Gergely et al., 2007 ). We therefore argue that higher levels of cumulative culture depend on representational abilities, to be examined in detail below.
From Informative to Normative Conformity
A more recent line of argument for a qualitative difference between ape and human culture is based on the notion of ‘conformity’ ( Whiten et al., 2005 ; Claidière and Whiten, 2012 ; van de Waal et al., 2013 ; van Leeuwen and Haun, 2013 ). The term was originally defined as the alignment of one’s attitude with a majority position ( Asch, 1956 ; Cialdini and Goldstein, 2004 ), a ‘majority influence’ ( van Leeuwen and Haun, 2013 ). Recent studies suggest that conformity-like phenomena may also exist in animals, even to the point of forsaking a pre-existing individual preference for the majority’s preference ( Whiten et al., 2005 ; Hopper et al., 2011 ; Claidière and Whiten, 2012 ; van de Waal et al., 2013 ). However, the underlying cognitive mechanisms of these behavioral effects are largely unknown, particularly whether animals are simply biased to select the choice of the majority ( informational conformity ) or whether this is the result of social awareness and a desire to conform to the group ( normative conformity ; Deutsch and Gerard, 1955 ; Claidière and Whiten, 2012 ; van Schaik, 2012 ).
Importantly, while both mechanisms occur in humans, there is currently no good evidence for normative conformity in animals. In humans, normative conformity is demonstrated if individuals are less likely to choose the behavioral variant of the majority in private than social contexts ( Deutsch and Gerard, 1955 ), a paradigm that to our knowledge has not yet been used with non-human primates. An open question remains how important majority influences really are in the transmission of animal behavior, as most empirical studies have not quantified differences in social transmission rates as a function of the number of available models ( van Leeuwen and Haun, 2014 ), and whether there really exists a disproportionate tendency to copy the majority in non-humans. Another good indicator for normative conformity is the punishment of individuals who deviate from social norms ( Hill, 2009 , p. 276). In the animal behavior literature, the term ‘punishment’ usually refers to a retaliatory action that leads to future compliance by the punished individuals ( Clutton-Brock and Parker, 1995 ). Certain processes are shared by both informational and normative conformity ( van Schaik, 2012 ), with informational conformity forming the basis for normative conformity. A graded integration of already present underlying mechanisms, such as informational normativity, fairness-related behaviors ( Brosnan, 2013 ) or punishment, may have thus led to normative conformity.
Similar to what has been argued for cumulative culture, graded cognitive differences may explain the jump from informational to normative conformity. Normativity requires some representation of norms and its more complex expressions therefore will also depend on the extent to which representations can be stored, manipulated and compared ( Kaufmann and Clément, 2014 ). This leads to the suggestion that, from a proximate mechanistic perspective, the ability to access the representational content of one’s knowledge may represent the critical difference between humans and other species. The analysis of the representational dimension of culture requires a cognitive approach, which we will develop in the next sections.
A Cognitive Approach to the Study of Culture
Psychological studies of humans have repeatedly documented how culture affects cognition ( Mesquita and Frijda, 1992 ; Greenfield, 1997 ; Kitayama et al., 2003 ; Sperber and Hirschfeld, 2004 ; Nisbett and Miyamoto, 2005 ) in domains as diverse as spatial cognition (e.g., Levinson, 1992 ; Levinson et al., 2002 ), behavioral economics ( Henrich et al., 2005 ), or time perception ( Casasanto, 2008 ). For instance, children initially prefer geocentric (absolute) strategies in spatial memory tasks but by age eight show culturally dependent strategies, which is also reflected in their spatial language ( Haun et al., 2006 ). Additionally, the same study showed that great apes also prefer geocentric strategies, suggesting a shared evolutionary origin. However, despite such studies and despite considerable interest in the cognitive underpinnings of animal social behavior ( Call and Santos, 2012 ), less work has been conducted to understand how cognition and culture intertwine when it comes to representing knowledge in non-humans. As a result, the human-animal gap remains wide, with animal cultures characterized by group-specific catalogs of behaviors and human cultures characterized by group-specific catalogs of norms and their practices.
Nonetheless, one often quoted definition of culture in the animal culture debate is “the way we do things” ( McGrew, 2004 ). This requires an ability not only to mentally represent behaviors, but also to identify the majority default behavior and compare this with one’s own behavior. Humans are certainly endowed with the ability to analyze their and others’ behavior, which enables them to represent what they and others know and to define themselves in terms of cultural groups. We can define this ability as ‘thinking culturally’; but is there any indication for this in apes? Most animal studies have not attempted to address the extent to which mental representations affect cultural behavior.
One way to address the cognitive processes underlying animal culture empirically is to present individuals belonging to different cultural groups with a problem that can be solved in different ways. If the problem is solved in line with pre-existing behavioral preferences, then this can be interpreted as a signal for differences in underlying mental representations. This interpretation is particularly compelling when individuals do not seem to comprehend their environment in the same way, notably if one object (such as a stick) appears to be understood as a tool in one given community, but not in the other one. Possessing mental representations defines the ability to think ( Byrne, 1995 ); being able to access and modify these representations is a crucial feature to cope with everyday tasks. However, species may differ in their capacity to do so. In a recent example, two groups of chimpanzees in Uganda, the Sonso community of Budongo Forest and the Kanyawara community of Kibale Forest, were exposed to an identical problem, honey trapped in a cavity of a large tree trunk (Figure 1 , Gruber et al., 2009 ). The two communities differ culturally, especially in terms of whether or not they use sticks as foraging tools ( Whiten et al., 2001 ). Results showed that members of the two communities solved the problem with group-specific techniques consistent with their cultural knowledge, that is, stick use in Kanyawara and leaf-sponging in Sonso. Hence, the chimpanzees applied previously acquired tool use behavior to a novel foraging problem. A particularly relevant point was that, although all Kanyawara chimpanzees knew how to manufacture leaf-sponges, no one chose this technique.
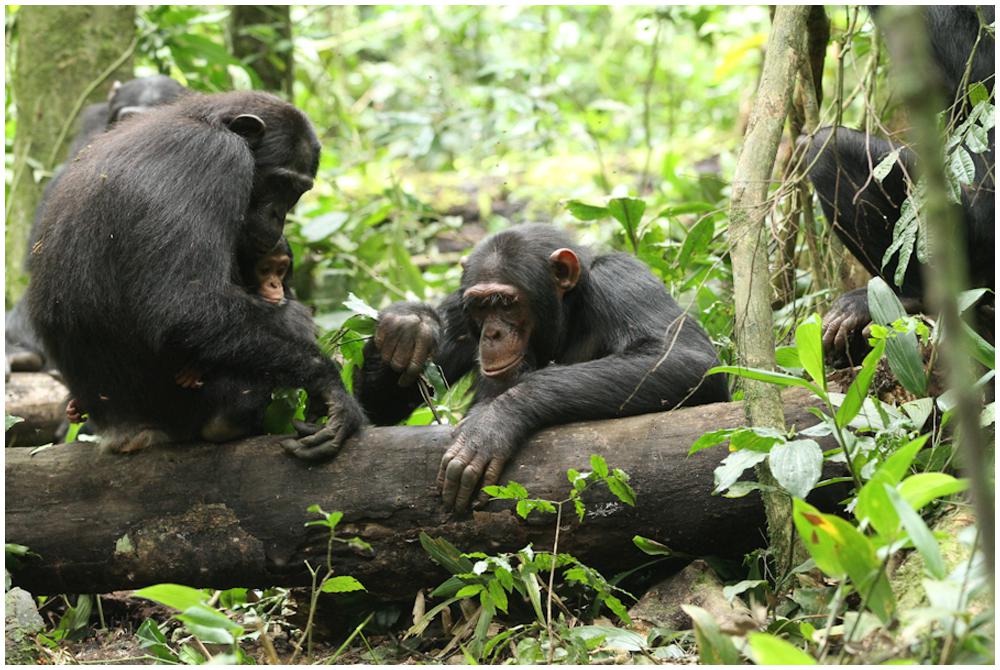
FIGURE 1. A member of the Kanyawara community extracting honey from the honey-trap apparatus during an experimental trial. (Kibale National Park, Uganda, Courtesy of Andrew Bernard).
In a follow-up experiment, individuals were exposed to the same problem, honey trapped in a cavity, but were also provided with a multi-functional tool, the ‘leafy-stick,’ which could be transformed into at least three different tools: a sponge, stick, or brush. Results showed that members of the two communities found different parts of the tool salient and used them accordingly. At Sonso, individuals detached the leaves from the provisioned tool to manufacture a leaf-sponge, while at Kanyawara, the chimpanzees used the stick part of the tool to dip for honey ( Gruber et al., 2011 ).
Overall, these experiments suggest that chimpanzee behavior is determined by previous experience, or knowledge, which can differ between communities ( Gruber et al., 2009 ). As a result, different communities may differ in how they recognize and use the affordances of an identical tool, suggesting that the way chimpanzees perceive their environment is biased by cultural knowledge ( Gruber et al., 2011 ).
In a related study, rehabilitant orang-utans (wild-born ex-captive individuals living in a sanctuary before reintroduction into the wild) were exposed to the same honey-dipping task and to a raking task. When individuals from two genetically distinct populations were tested they showed no difference in their performance in the raking task, suggesting that their potential understanding of sticks as tools was similar. In contrast, in the honey-dipping task, their performance varied in line with whether or not stick use was prevalent in their native populations, thus replicating the findings in chimpanzees ( Gruber et al., 2012b ). Recently, the same patterns have been reported from two populations of capuchin monkeys ( Sapajus apella ): monkeys that were naturally unfamiliar with manufacturing stick tools ignored and even discarded stick tools that were provisioned to gain access to an experimentally provided food source, while capuchins familiar with stick tool manufacture used sticks to obtain the same food (Ottoni, personal communication). Overall, these results are consistent with the view that, across primates and including humans, cultural knowledge influences the way individuals perceive their environment and how they act on it, in line with the notion of ‘cultural affordances,’ the “opportunities for perception and action offered by the environment to an organism” ( Kaufmann and Clément, 2007 , p. 226).
In spite of these results, an important unresolved question still is whether the mental representations underlying such variations are akin to ‘cultural ideas’ (or cultural mental representations), that is, mentally “stored form[s] derived from experience […] used to generate actions,” ( Bryson, 2009 , p. 83). Here, we define a mental representation as an internal cognitive construction of the mind that represents an aspect of the world . In doing so we follow Leslie (1987 , p. 414) who assumes that “…the basic evolutionary and ecological point of internal representation must be to represent aspects of the world in an accurate, faithful and literal way, in so far as this is possible for a given organism. Such a basic capacity for representation can be called a capacity for primary representations. Primary representation is thus defined in terms of its direct semantic relation with the world.” For instance, in the context of tool use, the idea of ‘stick use’ could be defined as a mental representation that contains the object ‘stick’ and some of its functional properties, which are defined in terms of specific actions. In other words, a learned association between a tool and a reward can be represented as a unique mental representation (e.g., ‘stick-to-get-honey,’ Figure 2A ). This mental representation can be cultural because it can be wildly shared within the members of a given community, as the behavior it represents ( Sperber, 1996 ).
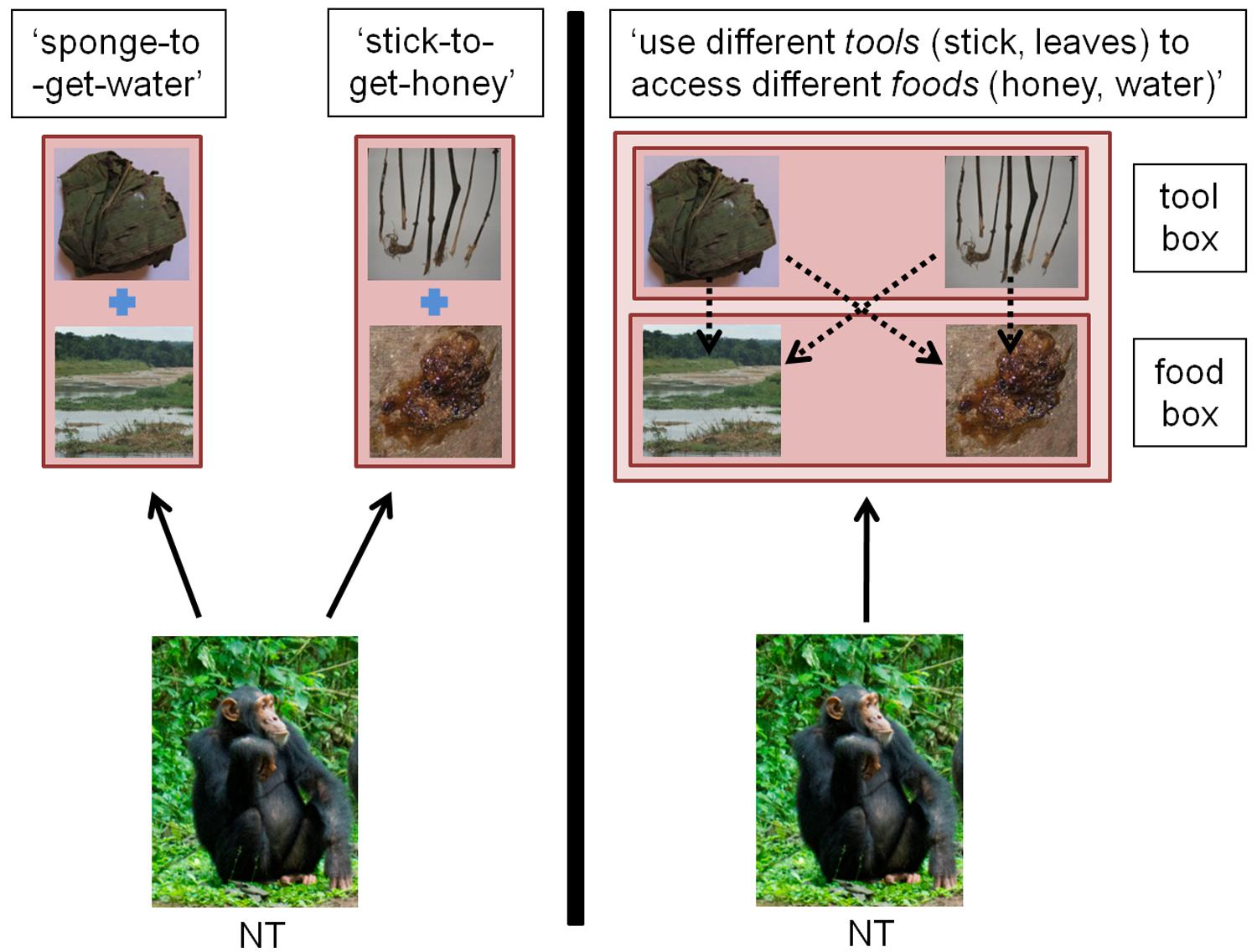
FIGURE 2. Comparison of a representational system where individuals build independent representations (A) or can re-organize their knowledge into categories (B) in the case of tool use . Full arrows: act of mentally representing. Square: content of mental representation, with or without embedded representations. Dashed arrows: connections within or between mental representations. (A) Independent Representations : individual NT forms a learned association between distinct parts of the environment (for example, a stick is associated with obtaining honey; a leaf-sponge is associated with obtaining water). The resulting two mental representations are independently stored in the individual’s mind. (B) Re-organization of knowledge in categories : individual NT organizes individual representations hierarchically, potentially under larger object kinds. For instance, ‘sponges’ and ‘sticks’ become members of the more general concept ‘tools’ in the individual’s own representational system and can be used interchangeably onto the different substrates ‘honey’ and ‘water.’ In the wild, chimpanzees are known to use leaf-sponges to fetch water, a behavior present in all studied communities. Additionally, in some communities, chimpanzees have been observed to use sticks to access liquid, a behavior named ‘fluid-dip’ ( Whiten et al., 2001 ). (Photos of honey, stick, and river by Thibaud Gruber; photos of chimpanzees and leaf-sponge, courtesy of Nina Hänninen and Cat Hobaiter).
Great apes are cognitive animals ( Call and Tomasello, 2008 ), in the sense that they can store their knowledge as primary representations, but the key question is whether they also have more complex representations, for instance, to represent and classify an object (e.g., stick) as belonging to a broader class (e.g., tool; Figure 2B ), and not solely attached to a given reward as in the ‘stick-to-get-honey’ mental construction. In the following, we apply conceptual tools of developmental psychology to analyze the complexity of mental representations underlying great ape cultural behavior.
Mental Representations of Artifacts: A Developmental Perspective
Developmental psychology has long been interested in how infants come to understand their environment and the objects found therein ( Piaget, 1929 ), including artifacts ( Margolis and Laurence, 2007 ). ‘Artifacts’ are a special class of objects because they have been modified or created for a specific purpose, and are thus major components of human material culture. Two-year-olds appear to understand object kind and some artifactual properties (for instance, they give an appropriate response to ‘a’ tool or ‘a’ musical instrument) but do not yet have an overall concept of tools ( Mandler, 2007 ). Three-year-olds, however, start to understand that artifacts belong to higher-order units, characterized by the purpose they are ‘made for’ ( DiYanni and Kelemen, 2008 ). This important cognitive and representational shift, which occurs between age five and seven, is characterized by a transition from a vague to a well-defined understanding of an artifact’s function and typical or intended use ( Defeyter and German, 2003 ).
Empirically, this shift can be demonstrated by what has been termed ‘ functional fixedness’ : children experience difficulties in solving a problem because of interference by previous knowledge. For instance, children may fail to see a solution to a tool-use problem if they are being offered a tool presented in a situation where it already has a well-defined purpose but where the situation requires a different use of this tool ( Defeyter and German, 2003 ). That children before age five do not show functional fixedness may be because they do not represent the intentionality of the maker, failing to understand that a tool has been intentionally manufactured by a designer to fulfill a specific function, a phenomenon known as adopting a design stance ( German and Defeyter, 2000 ; Kelemen and Carey, 2007 ). However, other interpretations of functional fixedness exist and do not connect it to the design stance. Individuals may simply fail to see multiple uses of an object because previous experience has led them to form an association between an object and a given function. Hence, the function itself is not represented as ‘intended.’ This interpretation has been argued for captive chimpanzees ( Hanus et al., 2011 ) and may explain why in the honey-trap experiments discussed before, chimpanzees failed to use sticks, mainly because this material is used daily to build nests, which may have prevented them from considering sticks as tools to extract honey. This interpretation obviously makes functional fixedness a less cognitively complex mechanism, but other wild chimpanzee communities have overcome any fixedness on nest-building by having learned to incorporate sticks into their extractive tool repertoire. This observation argues against the ‘simple’ functional fixedness hypothesis. This idea faces another problem when applied to the honey-trap experiment. It is unable to explain how the Sonso chimpanzees disregarded their only known function of leaf-sponges (to absorb drinking water) in favor of extracting honey from experimental cavities ( Gruber et al., 2011 ). This suggests that leaf-sponges are not functionally fixed to the purpose of extracting water, although experiments presenting water and honey simultaneously are needed to support this hypothesis. It is unlikely that the chimpanzees simply mistook the honey for water because it was very obvious during the experiments that subjects were aware that the resource was honey and not water, often visibly reacting to the stickiness of honey by rubbing their hands on the logs. Moreover, no individuals at Kanyawara made a sponge to extract the honey, despite leaf-sponging being customary in this community, suggesting that the confusion hypothesis can be rejected. Therefore, it is more plausible that the Sonso chimpanzees produced leaf-sponges to extract honey by some form of analogical reasoning ( Gillian et al., 1981 ), a cognitive process that requires access to representational content ( Gentner and Markman, 1997 ): they may either have considered that all liquids should be treated the same, or, reversely, that a leaf-sponge may be used on different liquids.
In summary, functional fixedness remains a possible explanation for the patterns observed in wild and captive chimpanzees, although it is difficult to decide whether this is based on simple or complex processes. Thus, it remains possible that chimpanzees access their mental representations in a more active way, akin to early reports of ‘insight’ ( Köhler, 1925 ). At the very least, individuals must have activated a mental representation (e.g., leaf-sponge) without the corresponding real world experience that generated the representation in the first place (waterhole). For example, a chimpanzee may think of a leaf-sponge when finding a valuable resource in a cavity, without seeing an actual leaf-sponge – it can start looking for the appropriate leaf material to manufacture one as a consequence. Accessing knowledge, however, may be cognitively more complex and may require that the subject also knows that it has the knowledge of leaf-sponges, which requires the ability to generate representations of representations, i.e., metarepresentations ( Sperber, 2000b ). In conclusion, while ‘simple’ functional fixedness appears to act without actively accessing representations (that is, individuals do not need to be aware of the content of their knowledge), ‘complex’ functional fixedness, as seen in older children, is based on representing intentions (a design stance), a form of metarepresentation.
To facilitate progress regarding the relation between chimpanzee and human culture, we will next survey the different levels of representation that may or may not underlie ape cultural behavior. Our focus will be on processes that can be described as ‘metarepresentational’ in the context of culture.
Metarepresentations and Culture
The ability to attribute psychological states to others and to oneself, or to have a ‘theory of mind,’ has been identified as the most important cognitive difference between humans and animals, including great apes ( Premack and Woodruff, 1978 ; Call and Tomasello, 2008 ). The classic test for investigating an individual’s theory of mind is the ‘Sally–Anne’ test, a false-belief task. In its standard version, the subject is presented with a performance during which an agent (a doll) witnesses how an object is being placed in box A, but then is prevented from seeing how the object is moved to another box B. As a result, the agent will have a false belief about where the object is hidden, i.e., in box A rather than box B. Human infants generally understand such tasks from age four ( Doherty, 2008 ), although more recent research has shown that precursor abilities required to solve the task emerge as early as age two ( Baillargeon et al., 2010 ).
Having a theory of mind requires some form of metarepresentational ability, the capacity to generate a representation of a representation. There is an ongoing debate about what exactly should qualify as a metarepresentation and different authors have proposed different terminologies. One important distinction has been to conceptualize metarepresentations either as ‘representations of representations’ (sense 1; e.g., Leslie, 1987 ) or as ‘representations of representations as representations’ (sense 2; e.g., Perner, 1991 ). In the latter case, the agent engaging in metarepresentation must represent the fact that whatever is represented is itself a representation. The paradigmatic example is the false-belief task, where the subject must understand that the agent’s representation of the world is ‘only’ a belief (object in box A), which differs from reality (object in box B). However, others have suggested that the subject does not necessarily have to be aware of the representational nature of the representation ( Leslie, 1987 ). Perner (1991) uses the term ‘ secondary representation ’ to refer to sense 1 metarepresentations, as opposed to sense 2 metarepresentations, or ‘true’ metarepresentations.
Whiten (2000) has proposed a useful terminological distinction between the two senses, keeping the name ‘metarepresentation’ for sense 2 and describing sense 1 as ‘re-representations.’ In doing so, he uses a term introduced by Karmiloff-Smith (1992) as a cognitive mechanism that allows accessing and sorting acquired information: “...a way to gain knowledge is for the mind to exploit internally the information that it has already stored (both innate and acquired), by redescribing its representations or, more precisely, by iteratively re-representing in different representational formats what its internal representations represent” (ibid., pp. 15–16). This individual-centered definition was subsequently extended to social processes by Whiten (2000) , who found evidence that enculturated apes engage in ‘re-representation’ during imitation and pretense. In sum, the term ‘re-representation’ (sense 1 metarepresentation, secondary representation) commonly describes a metarepresentation that does not require its owner to be aware of the representational nature of its representation, while the term ‘metarepresentation’ (sense 2 or true metarepresentation) requires an awareness of the representational nature (Table 1 ), a terminology that we also adopt in this paper. Additionally, the wording ‘metarepresentational processes’ will describe the general ability to go beyond simple representations, that is to engage at least in sense 1, and possibly in sense 2 metarepresentations.
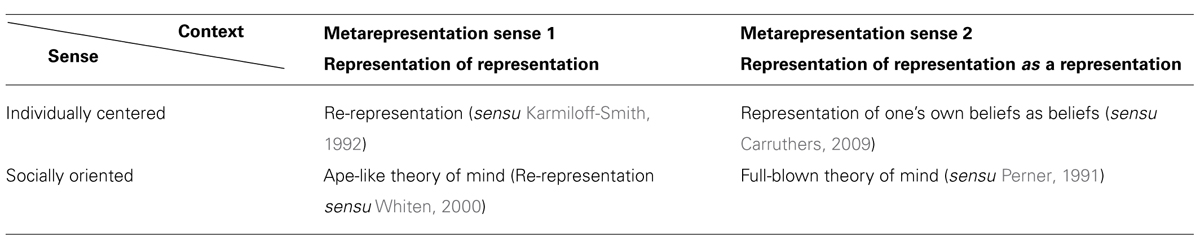
TABLE 1. Connection between Metarepresentation Sense 1 and Sense 2, the context, individually centered or socially oriented, in which they occur; and the way they have been described in the literature.
In the following section, we review the different metarepresentational processes which appear central to the representation of tools, and more generally to culture, and order them in a way that could constitute an evolutionary pathway. Our goal is to identify the different types of representations and metarepresentations that could underlie and sustain animal cultures 1 .
Re-Representations to Facilitate Categorisation and Conceptualisation
Group differences in tool use behavior importantly contributed to the claim that chimpanzees have culture ( Whiten et al., 1999 ). Nevertheless, it is still unclear whether chimpanzees and other tool-using primates resemble human infants ( Träuble and Pauen, 2007 ) in having a true understanding of ‘kinds,’ such as tools, or types of tools, such as hammers ( Hauser and Santos, 2007 ; Hernik and Csibra, 2009 ). Therefore, understanding how primates mentally represent tools is key for any comparison between human and ape cultures. Humans are often considered unique in their ability to represent objects as ‘made for something,’ that is, to naturally adopt a teleological stance when dealing with them ( Gergely and Csibra, 2003 ; Ruiz and Santos, 2013 ), an ability that appears to facilitate the acquisition of tool use behavior by toddlers ( Hernik and Csibra, 2009 ).
Not much theoretical work of this kind has been done in animals, despite the fact that great apes and capuchins are promising species to investigate these questions. Jackendoff (1989) argued that possessing a ‘true’ concept of something requires the ability to verbalize it. By this criterion, primates clearly lack the concept of ‘tool,’ but Jackendoff’s (1989) criterion may be unnecessary. In all likelihood, modern human language is a fairly recent evolutionary invention that emerged well after humans had developed complex and variable tools ( Mithen, 1996 ). Thus, a conceptual system of images, which may also be available to non-linguistic species, may well have preceded a conceptual system of words ( Gärdenfors, 2006 ).
An important question is whether animals can represent tools at a conceptual level (that is representing tools as objects with a given function to act on other objects) and not solely at a perceptual level (that is representing a tool based on its physical properties, Mandler, 2000 ). For example, can a chimpanzee categorize a leaf-sponge not only in terms of its perceived features (wadge of folded leaves) but also in terms of its function or purpose (liquid-absorption)? One possible way to investigate this question is to study whether apes classify novel objects according to functional (i.e., intended use) or perceptual similarities with familiar objects, similar to earlier paradigms developed to study analogical reasoning in chimpanzees ( Gillian et al., 1981 ). Work with cotton-top tamarins and rhesus monkeys has shown that individuals can group objects into meaningful categories, such as tools, foods, animals or landmarks, as well as recognize distinctive features of tools (see Hauser and Santos, 2007 for a review). And for New Caledonian crows, it has already been shown that individuals can sort objects according to function, e.g., as sinking versus floating devices ( Taylor et al., 2011 ; Jelbert et al., 2014 ). Considering these results, it appears likely that tool-using primates such as chimpanzees, orang-utans or capuchins represent their tools as particular objects with a function to act on other parts of their environment, that is, at the conceptual level, but experimental work is needed to confirm this hypothesis.
The main benefit of re-representations is that they allow their bearer to reorganize acquired information, for example by allocating objects to categories, such as a leaf-wadge to a sponge tool. Figure 2 illustrates this process in the context of tool use as a shift from a simple to a complex representational format. In the simple representational format, each tool is mentally represented as having one purpose (e.g., ‘sponge-to-get-water’) with no connection between representations. In the complex representational format, simple representations also belong to more general categories, and the items belonging to one category can be selected to function on the items belonging to a different category [e.g., ‘use different tools (stick, leaves) to access different foods (honey, water)’]. One relevant observation here is that in the Gruber et al. (2011) study, the Sonso chimpanzees spontaneously used leaf-sponges to extract honey, although this tool is widely used by wild chimpanzees for no other purpose than to extract water from streams or cavities ( Whiten et al., 1999 ). One interpretation of this finding is that leaf-sponges are not exclusively and rigidly represented in connection with water, suggesting that the Sonso chimpanzees have employed re-representational abilities to find this solution. Nonetheless, because they appear to fail to consider sticks as potential tools in other experiments, their re-representational abilities may only allow some flexibility around already known artifacts but may be too limited to generate the general concept of ‘tool.’
Re-Representations as Representations of Techniques
A second characteristic of re-representations is to allow an individual to maintain multiple mental representations simultaneously. During imitation, for instance, an individual may hold representations of an action’s desired outcome and an effectively executed motor pattern to achieve it ( Whiten, 2000 ). Similarly, an individual may be able to simultaneously maintain separate mental models of two actions in order to compare them ( Perner, 1991 ). In the case of ape tool use, for example, re-representations may allow an individual to generate representations of competing techniques and compare them to solve a problem (e.g., representations of leaf-sponging and stick-using to obtain honey; Figure 3A ). A recent study from the Sonso chimpanzee community is in line with this interpretation. In November 2011, a few individuals discovered a novel tool behavior, moss-sponging, to access water from a natural clay hole ( Hobaiter et al., 2014 ). Importantly, all moss-using individuals were already skilled leaf-sponge makers, suggesting that they possessed mental representations of two techniques for one outcome, accessing clay water, or that they modified their existing mental representation of a leaf-sponge to add the possibility of moss instead of leaves, in contrast to others who did not develop the novel behavior. Whether or not individuals also compared both representations cannot be decided by this study. Interestingly, the two techniques differ in efficiency (moss-sponges appear to hold water better than leaf-sponges), suggesting that individuals should prefer moss-sponging, whenever moss is locally available. Primates are capable of assessing the physical properties of their tools, in particular with respect to size and weight of potential objects that can be used as tools to complete a task ( Matsuzawa, 1994 ; Fox et al., 1999 ; Visalberghi et al., 2009 ). However, there is also evidence for cultural conservatism and individual habit formation in primates, which may prevent them from changing techniques ( Hrubesch et al., 2009 ; Gruber et al., 2011 ; Brosnan and Hopper, 2014 ). More field experiments are needed to address how chimpanzees and other animals evaluate the efficiency of their techniques. If chimpanzees choose a solution that is more efficient than a habitual technique already present in their repertoire, a stronger association between the novel tool and the original substrate may be formed, leading ultimately to a change in the tool choice. However, this may require several trials to be achieved, which may not always be granted in natural settings. The ability to compare mental representations, allowed by re-representational abilities, may allow switching to the novel technique directly after one individual trial or after witnessing others display this technique during social learning, making it a much faster, potentially more adaptive, process.
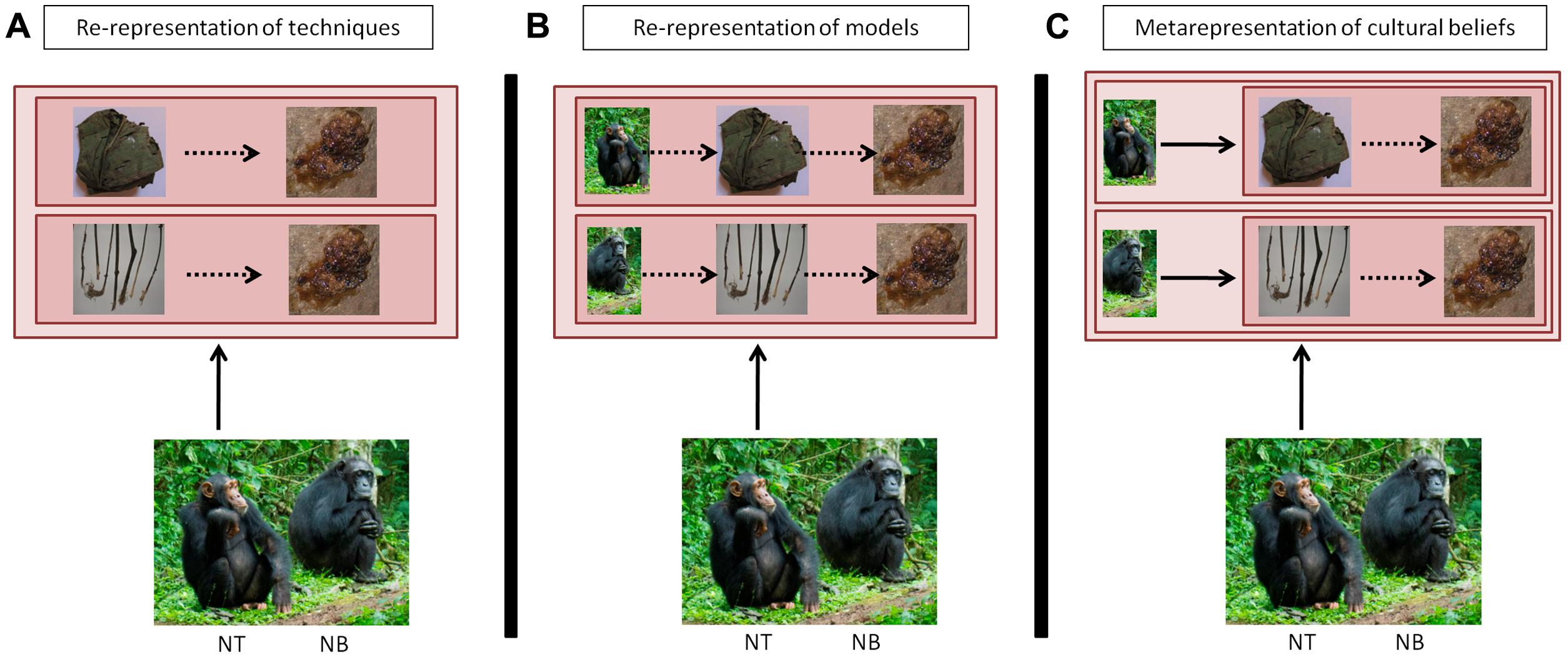
FIGURE 3. Comparison of a representational system where individuals can re-represent in parallel several actions in their mind (A); can re-represent the identity of the individual attached to the representation of the tool-using activity (B); and can fully metarepresent that other individuals may have different beliefs than themselves, applied to tool use (C) . Full arrows: act of mentally representing. Square: content of mental representation, with or without embedded representations. Dashed arrows: connections within or between mental representations. (A) Re-representations of techniques : individual NT represents in parallel two different ways to achieve the same outcome: ‘obtaining honey.’ Re-representations allow individual NT to access and compare both techniques, to select the most suitable one to achieve the task. (B) Re-representation of techniques displayed by individuals : individual NT represents in parallel that she is obtaining honey by using a leaf-sponge, while individual NB is obtaining the same resource with a different tool, a stick. (C) Metarepresentation of others’ cultural beliefs : here, individual NT knows that she has certain beliefs, for instance that honey should be gathered with a leaf-sponge; she also knows that other individuals such as NB possess systems of beliefs – that is, individual NT represents that individual NB represents that… – and also represents the fact that the content of these beliefs may be similar or different from her own. In the illustrated case, individual NB knows that the best way to obtain honey is by using a stick, which differs from NT’s information, which may result from individual or cultural learning. (Photos of honey, stick and river by Thibaud Gruber; photos of chimpanzees and leaf-sponge, courtesy of Nina Hänninen and Cat Hobaiter).
Re-Representations as Representation of Individual Differences
A more complex form of re-representation is for an individual to understand that it is carrying out one technique while others may carry out the same or a different technique, albeit toward the same goal; or the individual may also represent itself performing two different techniques. Here, the re-representation is not only functional but also has a social dimension, by means of comparing the self with others or between others. This mechanism may underlie the perception of intergroup differences, if such observations are possible. In chimpanzees, this will be rarely the case because of the species’ intense intergroup hostility ( Herbinger et al., 2009 ; Wilson et al., 2014 ) but this may be studied in dispersing adolescent female chimpanzees who adopt their new community’s cultural practices ( Luncz and Boesch, 2014 ). It would also be interesting to investigate how bonobos, who tend to be much more tolerant to the presence of strangers ( Furuichi, 2011 ), would react to the sight of other communities displaying different behaviors from themselves. One prediction thus is that normative aspects of culture will only appear in species where close intergroup interactions are common, a necessary precondition for the evolution of group-mindedness as a psychological trait ( Gruber and Zuberbühler, 2012 ; Tomasello et al., 2012 ). Equally relevant are imitation tasks, in which chimpanzees of high social status tend to be preferred as models for new techniques; however, this may also result from the fact that they are generally more attended to ( Horner et al., 2010 ; Kendal et al., 2014 ). In Hobaiter et al.’s (2014) study, social learning was very effective for moss-sponging, first shown by the alpha male, but not very effective for leaf-sponge re-use, first shown by lower-ranking individuals. Overall, these findings suggest that information on the identity of the model is part of an individual’s representation of a technique (Figure 3B ).
Re-Representations as a Basis for the Cumulativeness Aspect of Culture
A final characteristic of re-representations is that they allow representing tools, techniques, and the function of each component or sequence of actions ( Byrne and Russon, 1998 ) and their outcomes. They therefore allow representing a tool independently from the functional scheme in which it was originally defined. The ability to hold several representations in one’s mind at the same time and compare them may help an individual to associate different parallel functional schemes with complementary outcomes, thus leading to the creation of a novel scheme combining the two former ones. For instance, to build a composite tool, it is necessary to represent that both components can afford the same action, but also that their association results in higher efficiency. The Acheulean hand-ax, observed from about 1.7 million years ago, may have required well developed re-representational processes to form the necessary mental template ( Mithen, 2007 ; McPherron, 2013 ).
The near-absence of cumulative culture in apes, may therefore be due to their limited re-representational abilities, allowing some flexibility around behaviors that are already present in the repertoire (for instance to invent a moss-sponge based on a known leaf-sponge) but making qualitative jumps very unlikely. In contrast, belief-based metarepresentations do not seem crucial to analyze the functional schemes present in one’s current knowledge and to seek how to improve them. Re-representations may also sustain other complex cognitive processes recently proposed to be involved in the cumulativeness of human culture such as mental time travel ( Fogarty et al., 2012 ; Vale et al., 2012 ). There is evidence for mental time travel coming from a range of other animals than humans, including great apes and corvids (e.g., van Schaik et al., 2013 ), although alternative explanations have been proposed ( Fogarty et al., 2012 ; Vale et al., 2012 ). This suggests that some re-representational abilities are present in these species but that their extent is limited. In sum, more work is needed to precisely understand the scope of re-representations and their use in animals.
Metarepresentations to Represent Others’ Cultural Knowledge
The highest stage of metarepresentational process, in our context, is to appreciate that members of another group may harbor beliefs that are different from one’s own group, that is, to compare ‘how things ought to be’ (Figure 3C ). Here, cognition goes beyond simple re-representations, which could sustain all previous aspects of cultural knowledge, i.e., categorisation, representation of techniques, and representation of models. In effect, the metarepresentational processes must become ‘representations of representations as representations’ ( sensu Perner, 1991 , see Table 1 ), that is metarepresentations. In humans, this type of metarepresentation probably underlies complex mental state attribution, intentional teaching and belief-based imitation, the human ‘theory of mind’ ( Tomasello et al., 2005 and comments; Meltzoff, 2007 ). The ability to mentally represent and compare own and others’ knowledge may refine the categorisation of partners as ‘same’ or ‘other.’ Such reasoning, if associated with feelings of group identity, appears to be an ingredient for the emergence of social norms. Humans have an urge to conform to the behavior of others, but to perceive group behavior as normative and recognize deviation, it is also necessary to mentally represent the group norm, ‘the way things ought to be.’ Humans tend to become aggressive toward non-followers, while positive reinforcement also plays a role, for instance, in the case of the ‘chameleon effect,’ when individuals engaged in an interaction unintentionally match each other’s behaviors ( Chartrand and Bargh, 1999 ). How this effect connects to norms, however, remains to our knowledge to be investigated.
The theory of mind of great apes, in contrast, appears to be more limited and unable to take into account others’ false beliefs, suggesting that their metarepresentational abilities are equally limited ( Call and Tomasello, 2008 ). Chimpanzees have access to others’ perceptual knowledge ( Hare et al., 2000 , 2001 ), but appear to have great difficulties accessing others’ beliefs, especially if they deviate from their own ( Kaminski et al., 2008 ; Krachun et al., 2010 ). However, a research program studying how apes assess their own and others’ cultural knowledge has yet to be implemented. This research may also benefit other areas of metarepresentation. Controlled learning experiments (e.g., Marshall-Pescini and Whiten, 2008 ) will help to determine whether knowledgeable chimpanzees adjust their behavior to the state of knowledge of their naïve observers. During teaching, representing both the current state of knowledge of naïve individuals at the time of learning and the desired state of knowledge they must reach before being considered knowledgeable (e.g., they have acquired a set of rules or a technique) is crucial for knowledge transmission. In this respect, the ability to evaluate the state of knowledge of naïve observers, relying on metarepresentations, has most probably contributed to develop the full-fledge pedagogy now displayed by modern humans ( Csibra and Gergely, 2009 , 2011 ). While this remains to be tested, it is therefore unlikely that a chimpanzee would be shocked when it sees another behaving differently from the group norm, which would require the individual to understand that others may have values that deviate from its own and, by extension, to have a profound understanding of its own culture. Apes, in other words, are unlikely to consider whether ‘this is the right way to do it,’ a crucial feature of the human cultural mind and normative culture.
In sum, metarepresentations allow individuals to represent both one’s own cultural beliefs and those of others ( Sperber, 2000a ). Currently, we do not know whether this ability is present in apes and thus whether ape and human cultures differ in this important domain (see Table 2 ). As of now, the empirical data are consistent with what we call the ‘Jourdain Hypothesis,’ which states that apes have culture, but do not know that they do, in analogy to Molière’s Monsieur Jourdain, who had been speaking prose for 40 years without realizing it. By analogy, chimpanzees may practice their culture without knowing that they do, and future research must assess whether they can represent their own and others’ cultural behaviors as cultural norms. In the previous sections, we have shown that the ability to represent one’s own knowledge and others’ knowledge and the different ways of doing so appear fundamental to the human form of culture (e.g., Henrich et al., 2010 ). It thus appears necessary to determine whether a ‘Jourdain-like culture,’ that is, a culture its holder does not represent, can qualify as ‘culture,’ in particular with respect to other hominoids and to our earliest hominin ancestors, whose mental abilities may have been similar to those of great apes ( Mithen, 1996 ).
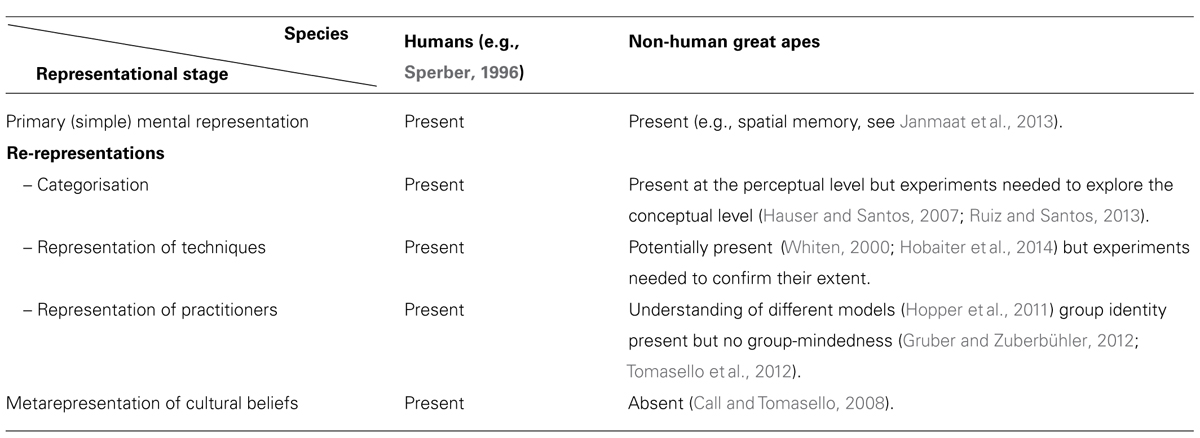
TABLE 2. Summary of the different stages of representations involved in the cultural process and their presence in humans and great apes, according to current knowledge.
Summary and Future Directions
The notion of metarepresentation has become widely used in false-belief research, while other metarepresentational processes have been neglected ( Sperber, 2000b ). Similarly, in the animal culture discussion, the latter have only played a role under the rubric of mindreading in comparing social learning processes that can lead to behavioral traditions, namely imitation and teaching ( Tomasello et al., 2005 and comments). In this article, we have argued that metarepresentational processes may be useful to explain a wider range of features of human and animal cultures, from representing objects as culture-specific, meaningful artifacts to understanding that another individual may or may not share a cultural belief. For animals, it is conceivable that social learning acting on innovations driven by the environment is sufficient to generate the full range of behavioral traditions currently documented, but several recent studies question this hypothesis ( van de Waal et al., 2013 ; Luncz and Boesch, 2014 ). In humans, however, culture is a co-construction of minds, and this may require considerable flexibility in how knowledge is organized. Therefore, culture without metarepresentational processes may never go beyond simple collections of behavioral traditions, acquired through social learning, usually confined within small social units (e.g., Hirata et al., 2001 ; Muller and Cant, 2010 ), and rarely spreading into group-specific patterns. In contrast, culture with simple metarepresentational processes (re-representation) may be present in great apes, and this may have served as the evolutionary origins of another type of culture: a pattern of ideas that have normative force. Finally, culture with complex metarepresentation characterizes human culture, which is based on belief psychology, shared knowledge, values, and norms.
In this article, we focussed largely on chimpanzee tool use to illustrate how to analyze the cognitive aspects underlying animal culture, particularly the role of mental representations. We believe that our framework has a generic value and can be applied to all species with behavioral traditions, granted that they have the necessary brain structures for higher cognition, such as a neocortex (mammals) or equivalent structures in other species (for instance, the dorsal ventricular ridge in birds, Dugas-Ford et al., 2012 ), following Gärdenfors (2006) . Although much of animal culture is material, there is evidence that a number of social behaviors also qualify as cultural (e.g., Whiten et al., 1999 ; Perry et al., 2003a ; van Schaik et al., 2003 ), suggesting they make equally interesting candidates to study the role of representations, re-representations and metarepresentations.
In conclusion, to properly compare animal and human cultures it is necessary to identify the metarepresentational processes that underlie behavior. We have identified two types of metarepresentational processes. The first one is self-oriented, allowing an individual to access its own knowledge (‘re-representation’). The second one is based on mental state attributions, allowing an individual to have access to more efficient transmission of knowledge (‘metarepresentation’). How the two co-evolved will need consideration, notably as primate cognition evolved within stable social groups ( Byrne and Whiten, 1988 ). Great apes are the only available model to assess the evolutionary transition in behavior and cognitive abilities from early hominins to modern humans ( McPherron, 2013 ). It seems safe to assume that early hominins possessed material cultures at least as complex as described for modern great apes ( van Schaik et al., 2003 ), but at what stage they also became aware of their own culture as one possible variant is impossible to decide. Progress can be made by targeted research on great apes and other animals, concerning their relationships with artifacts. A key test is whether an animal would be surprised if another group member deviated from an established technique to solve a familiar task. Current progress in infant and child cognition research offers promising new avenues, notably in adapting studies of object categorisation, representation, and conceptualisation ( Kelemen and Carey, 2007 ; Mandler, 2007 ; DiYanni and Kelemen, 2008 ; Ruiz and Santos, 2013 ). For instance, research on functional fixedness will provide a deeper understanding of the cognitive underlay of primate culture. Here, the key prediction is that chimpanzees raised in captivity (with no experience in nest building) should solve the honey-trap experiment more easily than wild chimpanzees with no stick use tradition (such as the Sonso community).
It is possible that great apes have more advanced metarepresentational capacities than generally thought ( Call and Tomasello, 2008 ), although they seem to be better described as re-representations ( sensu Karmiloff-Smith, 1992 ). Building on previous work, we argued that apes may be able to search through their own cultural knowledge to select the most adequate tool for a given task, as described in Figures 2B and 3A , but more research is needed to test this hypothesis. In particular, experiments are required in which apes need to maintain several representations simultaneously to succeed. While they are probably able to associate particular individuals with particular techniques (Figure 3B ), it seems less likely that they can associate a given behavior with a group of individuals, a necessary condition to understanding culture as a shared property of minds. Again, specific experiments will be required to address this hypothesis. Finally, we find it implausible that apes are able to attribute cultural beliefs to members of their groups (Figure 3C ), which would require complex metarepresentational abilities. Once the necessary studies have been conducted, it should be possible to draw more certain conclusions about the nature of the mental representations underlying animal culture, which is ultimately necessary to understand the evolution of the human cultural mind.
Conflict of Interest Statement
The authors declare that the research was conducted in the absence of any commercial or financial relationships that could be construed as a potential conflict of interest.
Acknowledgments
The research leading to these results has received funding from the People Programme (Marie Curie Actions) and from the European Research Council under the European Union’s Seventh Framework Programme for research, technological development and demonstration under REA grant agreement n°329197 awarded to Thibaud Gruber, ERC grant agreement n° 283871 awarded to Klaus Zuberbühler. We thank the Associate Editor and three anonymous reviewers for comments on earlier versions of the manuscript.
- ^ We argue that the ability to form metarepresentations enables an individual to engage in self-analyzing processes (see Table 1 ), an ability also termed ‘metacognition’ ( Beran et al., 2012 ). In animals, metacognition is typically studied with uncertainty tasks, in which a subject may opt for a lower-value reward when it is unsure to obtain a large reward depending on how correctly it can assess its own state of knowledge ( Beran et al., 2012 ). There is a debate on whether there is a true difference between metacognition and metarepresentation or whether the former is part of the latter. For instance, Carruthers (2009) regards metacognition as mindreading applied to the self (Table 1 ). The main point of debate is whether or not the content of metacognition is representational ( Proust, 2007 ; Carruthers, 2009 ). In our case, we have focussed on the representational nature of apes’ knowledge, suggesting that metarepresentation is the more appropriate term.
Allen, J., Weinrich, M., Hoppitt, and Rendell, L. (2013). Network-based diffusion analysis reveals cultural transmission of lobtail feeding in humpback whales. Science 340, 485–488. doi: 10.1126/science.1231976
Pubmed Abstract | Pubmed Full Text | CrossRef Full Text | Google Scholar
Asch, S. E. (1956). Studies of independence and conformity: I. A minority of one against a unanimous majority. Psychol. Monogr. Gen. Appl. 70, 1–70. doi: 10.1037/h0093718
CrossRef Full Text | Google Scholar
Baillargeon, R., Scott, R. M., and He, Z. (2010). False-belief understanding in infants. Trends Cogn. Sci. 14, 110–118. doi: 10.1016/j.tics.2009.12.006
Beran, M. J., Brandl, J., Perner, J., and Proust, J. (eds). (2012). Foundations of Metacognition. Oxford: Oxford University Press. doi: 10.1093/acprof:oso/9780199646739.001.0001
Biro, D., Inoue-Nakamura, N., Tonooka, R., Yamakoshi, G., Sousa, C., and Matsuzawa, T. (2003). Cultural innovation and transmission of tool use in wild chimpanzees: evidence from field experiments. Anim. Cogn. 6, 213–223. doi: 10.1007/s10071-003-0183-x
Boesch, C. (2013). “Ecology and cognition of tool use in chimpanzees,” in Tool Use in Animals: Cognition and Ecology , eds C. Sanz, J. Call and C. Boesch (Cambridge: Cambridge University Press).
Google Scholar
Boesch, C., Head, J., and Robbins, M. M. (2009). Complex tool sets for honey extraction among chimpanzees in Loango National Park, Gabon. J. Hum. Evol. 56, 560–569. doi: 10.1016/j.jhevol.2009.04.001
Brosnan, S. F. (2013). Justice- and fairness-related behaviors in non-human primates. Proc. Natl. Acad. Sci. U.S.A. 110, 10416–10423. doi: 10.1073/pnas.1301194110
Brosnan, S. F., and Hopper, L. M. (2014). Psychological limits on animal innovation. Anim. Behav. 92, 325–332. doi: 10.1016/j.anbehav.2014.02.026
Bryson, J. J. (2009). Representations underlying social learning and cultural evolution. Interact. Stud. 10, 77–100. doi: 10.1075/is.10.1.06bry
Byrne, R. W. (1995). The Thinking Ape: Evolutionary Origins of Intelligence . Oxford: Oxford University Press. doi: 10.1093/acprof:oso/9780198522652.001.0001
Byrne, R. W., and Russon, A. E. (1998). Learning by imitation: a hierarchical approach. Behav. Brain Sci. 21, 667–721. doi: 10.1017/S0140525X98001745
Byrne, R. W., and Whiten, A. (1988). Machiavellian Intelligence: Social Expertise and the Evolution of Intellect in Monkeys, Apes and Humans. Oxford: Oxford University Press.
Call, J., and Santos, L. (2012). “Understanding the minds of others,” in The Evolution of Primate Societies , eds J. Mitani, J. Call, P. Kappeler, R. Palombit, and J. Silk (Chicago: Chicago University Press), 664–681.
Call, J., and Tomasello, M. (2008). Does the chimpanzee have a theory of mind? 30 years later. Trends Cogn. Sci. 12, 187–192. doi: 10.1016/j.tics.2008.02.010
Carruthers, P. (2009). How we know our own minds: the relationship between mindreading and metacognition. Behav. Brain Sci. 32, 1–18. doi: 10.1017/S0140525X09000545
Casasanto, D. (2008). Who’s afraid of the Big Bad Whorf? Crosslinguistic differences in temporal language and thought. Lang. Learn. 58, 63–79. doi: 10.1111/j.1467-9922.2008.00462.x
Chartrand, T. L., and Bargh, J. A. (1999). The chameleon effect: the perception-behavior link and social interaction. J. Pers. Soc. Psychol. 76, 893–910. doi: 10.1037/0022-3514.76.6.893
Cialdini, R. B., and Goldstein, N. J. (2004). Social influence: compliance and conformity. Annu. Rev. Psychol. 55, 591–621. doi: 10.1146/annurev.psych.55.090902.142015
Claidière, N., and Sperber, D. (2010). Imitation explains the propagation, not the stability of animal culture. Proc. R. Soc. B Biol. Sci. 277, 651–659. doi: 10.1098/rspb.2009.1615
Claidière, N., and Whiten, A. (2012). Integrating the study of conformity and culture in humans and nonhuman animals. Psychol. Bull. 138, 126–145. doi: 10.1037/a0025868
Clutton-Brock, T. H., and Parker, G. A. (1995). Punishment in animal societies. Nature 373, 209–216. doi: 10.1038/373209a0
Csibra, G., and Gergely, G. (2009). Natural pedagogy. Trends Cogn. Sci. 13, 148–153. doi: 10.1016/j.tics.2009.01.005
Csibra, G., and Gergely, G. (2011). Natural pedagogy as evolutionary adaptation. Philos. Trans. R. Soc. B Biol. Sci. 366, 1149–1157. doi: 10.1098/rstb.2010.0319
Dean, L. G., Vale, G. L., Laland, K. N., Flynn, E., and Kendal, R. L. (2014). Human cumulative culture: a comparative perspective. Biol. Rev. 89, 284–301. doi: 10.1111/brv.12053
Defeyter, M. A., and German, T. P. (2003). Acquiring an understanding of design: evidence from children’s insight problem solving. Cognition 89, 133–155. doi: 10.1016/S0010-0277(03)00098-2
Deutsch, M., and Gerard, H. B. (1955). A study of normative and informational social influences upon individual judgment. J. Abnorm. Soc. Psychol. 51, 629–636. doi: 10.1037/h0046408
DiYanni, C., and Kelemen, D. (2008). Using a bad tool with good intention: young children’s imitation of adults’ questionable choices. J. Exp. Child Psychol. 101, 241–261. doi: 10.1016/j.jecp.2008.05.002
Doherty, M. J. (2008). Theory of Mind: How Children understand others’ Thoughts and Feelings . HoveTaylor & Francis Group.
Dugas-Ford, J., Rowell, J. J., and Ragsdale, C. W. (2012). Cell-type homologies and the origins of the neocortex. Proc. Natl. Acad. Sci. U.S.A. 109, 16974–16979. doi: 10.1073/pnas.1204773109
Fogarty, L., Rendell, L., and Laland, K. N. (2012). Mental time travel, memory, and the social learning strategies tournament. Learn. Motiv. 43, 241–246. doi: 10.1016/j.lmot.2012.05.009
Fox, E. A., Sitompul, A. F., and Van Schaik, C. P. (1999). “Intelligent tool use in wild sumatran orangutans,” in The Mentality of Gorillas and Orangutans , eds S. Parker, L. Miles, and A. Mitchell (Cambridge: Cambridge University Press), 99–117. doi: 10.1017/CBO9780511542305.005
Fragaszy, D. M., and Perry, S. (eds). (2003). The Biology of Traditions: Models and Evidence . Cambridge: Cambridge University Press. doi: 10.1017/CBO9780511584022
Furuichi, T. (2011). Female contributions to the peaceful nature of bonobo society. Evol. Anthropol. 20, 131–142. doi: 10.1002/evan.20308
Galef, B. G. (1992). The question of animal culture. Hum. Nat. 3, 157–178. doi: 10.1007/BF02692251
Galef, B. G. (2009). “Culture in animals?,” in The Question of Animal Culture , eds K. N. Laland and B. G. Galef (Cambridge, MA: Harvard University Press), 222–246.
Gärdenfors, P. (2006). How Homo became sapiens ? Oxford: Oxford University Press. doi: 10.1093/acprof:oso/9780198528517.001.0001
Gentner, D., and Markman, A. B. (1997). Structure mapping in analogy and similarity. Am. Psychol. 52, 45–56. doi: 10.1037/0003-066X.52.1.45
Gergely, G., and Csibra, G. (2003). Teleological reasoning in infancy: the naive theory of rational action. Trends Cogn. Sci. 7, 287–292. doi: 10.1016/S1364-6613(03)00128-1
Gergely, G., Egyed, K., and Király, I. (2007). On pedagogy. Dev. Sci. 10, 139–146. doi: 10.1111/j.1467-7687.2007.00576.x
German, T. P., and Defeyter, M. A. (2000). Immunity to functional fixedness in young children. Psychon. Bull. Rev. 7, 707–712. doi: 10.3758/BF03213010
Gillian, D. J., Premack, D., and Woodruff, G. (1981). Reasoning in the chimpanzee: I. Anal. Reason. J. Exp. Psych. Anim. Behav. Proc. 7, 1–17. doi: 10.1037/0097-7403.7.1.1
CrossRef Full Text
Greenfield, P. (1997). “Culture as process: empirical methods for cultural psychology,” in Handbook of Crosscultural Psychology, eds J. W. Berry, Y. H. Poortinga, and J. Pandey (Boston: Allyn & Bacon), 301–346.
Gruber, T., Muller, M. N., Reynolds, V., Wrangham, R. W., and Zuberbühler, K. (2011). Community-specific evaluation of tool affordances in wild chimpanzees. Sci. Rep. 1, 128. doi: 10.1038/srep00128
Gruber, T., Muller, M. N., Strimling, P., Wrangham, R. W., and Zuberbühler, K. (2009). Wild chimpanzees rely on cultural knowledge to solve an experimental honey acquisition task. Curr. Biol. 19, 1806–1810. doi: 10.1016/j.cub.2009.08.060
Gruber, T., Potts, K., Krupenye, C., Byrne, M.-R., Mackworth-Young, C., Mcgrew, W. C.,et al. (2012a). The influence of ecology on chimpanzee cultural behaviour: a case study of five Ugandan chimpanzee communities. J. Comp. Psychol. 126, 446–457. doi: 10.1037/a0028702
Gruber, T., Singleton, I., and Van Schaik, C. P. (2012b). Sumatran orangutans differ in their cultural knowledge but not in their cognitive abilities. Curr. Biol. 22, 2231–2235. doi: 10.1016/j.cub.2012.09.041
Gruber, T., and Zuberbühler, K. (2012). Evolutionary origins of the human cultural mind. Psychologist 25, 364–368.
Hanus, D., Mendes, N., Tennie, C., and Call, J. (2011). Comparing the performances of apes ( Gorilla gorilla , Pan troglodytes , Pongo pygmaeus ) and human children (Homo sapiens) in the floating peanut task. PLoS ONE 6:e19555. doi: 10.1371/journal.pone.0019555
Hare, B., Call, J., Agnetta, B., and Tomasello, M. (2000). Chimpanzees know what conspecifics do and do not see. Anim. Behav. 59, 771–785. doi: 10.1006/anbe.1999.1377
Hare, B., Call, J., and Tomasello, M. (2001). Do chimpanzees know what conspecifics know? Anim. Behav. 61, 139–151. doi: 10.1006/anbe.2000.1518
Haun, D. B. M., Rapold, C. J., Call, J., Janzen, G., and Levinson, S. C. (2006). Cognitive cladistics and cultural override in Hominid spatial cognition. Proc. Natl. Acad. Sci. U.S.A. 103, 17568–17573. doi: 10.1073/pnas.0607999103
Hauser, M. D., and Santos, L. R. (2007). “The evolutionary ancestry of our knowledge of tools: from percepts to concepts,” in Creations of the Mind: Theories of Artifacts and their Representation , eds E. Margolis and S. Laurence (Oxford: Oxford University Press), 267–288.
Henrich, J., Boyd, R., Bowles, S., Camerer, C., Fehr, E., Gintis, H.,et al. (2005). “Economic man” in cross-cultural perspective: behavioral experiments in 15 small-scale societies. Behav. Brain Sci. 28, 795–855. doi: 10.1017/S0140525X05000142
Henrich, J., Heine, S., and Norenzayan, A. (2010). The weirdest people in the world? Behav. Brain Sci. 33, 61–135. doi: 10.1017/S0140525X0999152X
Herbinger, I., Papworth, S., Boesch, C., and Zuberbühler, K. (2009). Vocal, gestural, and locomotor responses of wild chimpanzees to familiar and unfamiliar intruders: a playback study. Anim. Behav. 78, 1389–1396. doi: 10.1016/j.anbehav.2009.09.010
Hernik, M., and Csibra, G. (2009). Functional understanding facilitates learning about tools in human children. Curr. Opin. Neurobiol. 19, 34–38. doi: 10.1016/j.conb.2009.05.003
Heyes, C. M. (1998). Theory of mind in nonhuman primates. Behav. Brain Sci. 21, 101–148. doi: 10.1017/S0140525X98000703
Hill, K. (2009). “Animal ”culture”?,” in The Question of Animal Culture , eds K. N. Laland and B. G. Galef (Cambridge, MA: Harvard University Press), 269–287.
Hirata, S., Watanabe, K., and Kawai, M. (2001). “Sweet-potato washing” revisited,” in Primate Origins of Human Cognition and Behaviour , ed. T. Matsuzawa (Tokyo: Springer), 487–508.
Hobaiter, C., and Byrne, R. W. (2010). Able-bodied wild chimpanzees imitate a motor procedure used by a disabled individual to overcome handicap. PLoS ONE 5:e11959. doi: 10.1371/journal.pone.0011959
Hobaiter, C., Poisot, T., Zuberbühler, K., Hoppitt, W., and Gruber, T. (2014). Social network analysis shows direct evidence for social transmission of tool use in wild chimpanzees. PLoS Biol. 12:e1001960. doi: 10.1371/journal.pbio.1001960
Hopper, L. M., Schapiro, S. J., Lambeth, S. P., and Brosnan, S. F. (2011). Chimpanzees’ socially maintained food preferences indicate both conservatism and conformity. Anim. Behav. 81, 1195–1202. doi: 10.1016/j.anbehav.2011.03.002
Horner, V., Proctor, D., Bonnie, K. E., Whiten, A., and De Waal, F. B. M. (2010). Prestige affects cultural learning in chimpanzees. PLoS ONE 5:e10625. doi: 10.1371/journal.pone.0010625
Hrubesch, C., Preuschoft, S., and Van Schaik, C. P. (2009). Skill mastery inhibits adoption of observed alternative solutions among chimpanzees ( Pan troglodytes ). Anim. Cogn. 12, 209–216. doi: 10.1007/s10071-008-0183-y
Hunt, G. R., and Gray, R. D. (2003). Diversification and cumulative evolution in new caledonian crow tool manufacture. Proc. R. Soc. Biol. Sci. 270, 867–874. doi: 10.1098/rspb.2002.2302
Jackendoff, R. (1989). What is a concept, that a person may grasp it? Mind Lang. 4, 68–102. doi: 10.1111/j.1468-0017.1989.tb00243.x
Jaeggi, A., Dunkel, L., Van Noordwijk, M. A., Wich, S. A., Sura, A. A. L., and Van Schaik, C. P. (2010). Social learning of diet and foraging skills by wild immature Bornean orangutans: implications for culture. Am. J. Primatol. 72, 62–71. doi: 10.1002/ajp.20752
Janmaat, K. R. L., Ban, S. D., and Boesch, C. (2013). Chimpanzees use long-term spatial memory to monitor large fruit trees and remember feeding experiences across seasons. Anim. Behav. 86, 1183–1205. doi: 10.1016/j.anbehav.2013.09.021
Jelbert, S. A., Taylor, A. H., Cheke, L. G., Clayton, N. S., and Gray, R. D. (2014). Using the Aesop’s fable paradigm to investigate causal understanding of water displacement by new caledonian crows. PLoS ONE 9:e92895. doi: 10.1371/journal.pone.0092895
Kaminski, J., Call, J., and Tomasello, M. (2008). Chimpanzees know what others know, but not what they believe. Cognition 109, 224–234. doi: 10.1016/j.cognition.2008.08.010
Karmiloff-Smith, A. (1992). Beyond Modularity: A Developmental Perspective on Cognitive Science . Cambridge, MA: MIT Press.
Kaufmann, L., and Clément, F. (2007). How culture comes to mind: from social affordances to cultural analogies. Intellectica 46, 221–250.
Kaufmann, L., and Clément, F. (2014). Wired for society: cognizing pathways to society and culture. Topoï 33, 459–475. doi: 10.1007/s11245-014-9236-9
Kelemen, D., and Carey, S. (2007). “The essence of artifacts: developing the design stance,” in Creations of the Mind: Theories of Artifacts and their Representation , eds E. Margolis and S. Laurence (Oxford: Oxford University Press), 212–230.
Kendal, R., Hopper, L. M., Whiten, A., Brosnan, S. F., Lambeth, S. P., Schapiro, S. J.,et al. (2014). Chimpanzees copy dominant and knowledgeable individuals: implications for cultural diversity. Evol. Hum. Behav. 36, 65–72. doi: 10.1016/j.evolhumbehav.2014.09.002
Kendal, R. L., Custance, D., Kendal, J. R., Vale, G., Stoinski, T., Rakotomalala, N. I.,et al. (2010). Evidence for social learning in wild lemurs (Lemur catta). Learn. Behav. 38, 220–234. doi: 10.3758/LB.38.3.220
Kitayama, S., Duffy, S., Kawamura, R., and Larsen, J. T. (2003). Perceiving an object and its context in different cultures: a cultural look at new look. Psychol. Sci. 14, 201–206. doi: 10.1111/1467-9280.02432
Köhler, W. (1925). The Mentality of Apes. London: Trubner & Co, Ltd.
Krachun, C., Carpenter, M., Call, J., and Tomasello, M. (2010). A new change-of-contents false belief test: children and chimpanzees compared. Int. J. Comp. Psychol. 23, 145–165.
Krützen, M., Willems, E. P., and Van Schaik, C. P. (2011). Culture and geographic variation in orang-utan behaviour. Curr. Biol. 21, 1808–1812. doi: 10.1016/j.cub.2011.09.017
Lehner, S. R., Burkart, J. M., and Van Schaik, C. P. (2011). Can captive orangutans ( Pongo pygmaeus abelii ) be coaxed into cumulative build-up of techniques? J. Comp. Psychol. 125, 446–455. doi: 10.1037/a0024413
Leslie, A. M. (1987). Pretense and representation: the origins of “theory of mind.” Psychol. Rev. 94, 412–426. doi: 10.1037/0033-295X.94.4.412
Levinson, S. C. (1992). Language and Cognition: Cognitive Sequences of Spatial Description in Guugu Yimithirr (Working Paper No. 13). Nijmegen: Cognitive Anthropology Research Group, Max Planck Institute.
Levinson, S. C., Kita, S., Haun, D. B. M., and Rasch, B. H. (2002). Returning the tables: language affects spatial reasoning. Cognition 84, 155–188. doi: 10.1016/S0010-0277(02)00045-8
Luncz, L. V., and Boesch, C. (2014). Tradition over trend: neighboring chimpanzee communities maintain differences in cultural behavior despite frequent immigration of adult females. Am. J. Primatol. 76, 649–657. doi: 10.1002/ajp.22259
Luncz, L. V., Mundry, R., and Boesch, C. (2012). Evidence for cultural differences between neighboring chimpanzee communities. Curr. Biol. 22, 922–926. doi: 10.1016/j.cub.2012.03.031
Mandler, J. M. (2000). Perceptual and conceptual processes in infancy. J. Cogn. Dev. 1, 3–36. doi: 10.1207/S15327647JCD0101N_2
Mandler, J. M. (2007). “The conceptual foundations of animals and artifacts,” in Creations of the Mind: Theories of Artifacts and their Representation , eds E. Margolis and S. Laurence (Oxford: Oxford University Press), 191–211.
Margolis, E., and Laurence, S. (eds). (2007). Creations of the Mind: Theories of Artifacts and their Representation . Oxford: Oxford University Press.
Marshall-Pescini, S., and Whiten, A. (2008). Social learning of nut-cracking behaviour in East African sanctuary-living chimpanzees. J. Comp. Psychol. 122, 186–194. doi: 10.1037/0735-7036.122.2.186
Matsuzawa, T. (1991). Nesting cups and meta-tool in chimpanzees. Behav. Brain Sci. 14, 570–571. doi: 10.1017/S0140525X00071417
Matsuzawa, T. (1994). “Field experiments on use of stone tools by chimpanzees in the wild,” in Chimpanzee Cultures , eds R. W. Wrangham, W. C. Mcgrew, F. B. M. D. Waal, and P. G. Heltne (Cambridge, MA: Harvard University Press), 351–370.
McGrew, W. C. (2004). The Cultured Chimpanzee: Reflections on Cultural Primatology . Cambridge: Cambridge University Press. doi: 10.1017/CBO9780511617355
McPherron, S. P. (2013). “Perspectives on stone tools and cognition in the early Paleolithic record,” in Tool Use in Animals: Cognition and Ecology , eds C. M. Sanz, J. Call, and C. Boesch (Cambridge: Cambridge University Press), 286–309.
Meltzoff, A. N. (2007). ‘Like me’: a foundation for social cognition. Dev. Sci. 10, 126–134. doi: 10.1111/j.1467-7687.2007.00574.x
Mesquita, B., and Frijda, N. H. (1992). Cultural variations in emotions: a review. Psychol. Bull. 112, 179–204. doi: 10.1037/0033-2909.112.2.179
Mithen, S. (1996). The Prehistory of the Mind. London: Thames and Hudson.
Mithen, S. J. (2007). “Creations of Pre-modern human minds: stone tool manufacture,” in Creations of the Mind: Theories of Artifacts and their Representation , eds E. Margolis and S. Laurence (Oxford: Oxford University Press).
Muller, C. A., and Cant, M. A. (2010). Imitation and traditions in wild banded mongooses. Curr. Biol. 20, 1171–1175. doi: 10.1016/j.cub.2010.04.037
Nisbett, R. E., and Miyamoto, Y. (2005). The influence of culture: holistic versus analytic perception. Trends Cogn. Sci. 9, 467–473. doi: 10.1016/j.tics.2005.08.004
Perner, J. (1991). Understanding the Representational Mind. Cambridge, MA: Bradford books.
Perry, S. (2009). “Are nonhuman primates likely to exhibit cultural capacities like those of humans?,” in The Question of Animal Culture , eds K. N. Laland and B. G. Galef (Cambridge, MA: Harvard University Press).
Perry, S., Baker, M., Fedigan, L., Gros-Louis, J., Jack, K., Mackinnon, K. C.,et al. (2003a). Social conventions in wild white-faced capuchin monkeys: evidence for traditions in a neotropical primate. Curr. Anthropol. 44, 241–268. doi: 10.1086/345825
Perry, S., Panger, M., Rose, L., Baker, M., Gros-Louis, J., Jack, K.,et al. (2003b). “Traditions in wild white-faced capuchin monkeys,” in The Biology of Traditions: Models and Evidence , eds D. Fragaszy and S. Perry (Cambridge: Cambridge University Press).
Piaget, J. (1929). The Child’s Conception of the World . London: Routledge.
Pradhan, G. R., Tennie, C., and Van Schaik, C. P. (2012). Social organization and the evolution of cumulative technology in apes and hominins. J. Hum. Evol. 63, 180–190. doi: 10.1016/j.jhevol.2012.04.008
Premack, D., and Woodruff, G. (1978). Does the chimpanzee have a theory of mind? Behav. Brain Sci. 1, 515–526. doi: 10.1017/S0140525X00076512
Proust, J. (2007). Metacognition and metarepresentation: is a self-directed theory of mind a precondition for metacognition? Synthese 2, 271–295. doi: 10.1007/s11229-007-9208-3
Reader, S. M., and Biro, D. (2010). Experimental identification of social learning in wild animals. Learn. Behav. 38, 265–283. doi: 10.3758/LB.38.3.265
Rendell, L., and Whitehead, H. (2001). Culture in whales and dolphins. Behav. Brain Sci. 24, 309–324. doi: 10.1017/S0140525X0100396X
Ruiz, A. M., and Santos, L. R. (2013). “Understanding differences in the way human and non-human primates represent tools: the role of teleological-intentional information,” in Tool Use in Animals: Cognition and Ecology , eds C. M. Sanz, J. Call, and C. Boesch (Cambridge: Cambridge University Press), 119–133.
Samuni, L., Mundry, R., Terkel, J., Zuberbühler, K., and Hobaiter, C. (2014). Socially learned habituation to human observers in wild chimpanzees. Anim. Cogn. 17, 997–1005. doi: 10.1007/s10071-014-0731-6
Sanz, C. M., and Morgan, D. B. (2007). Chimpanzee tool technology in the Goualougo Triangle, Republic of Congo. J. Hum. Evol. 52, 420–433. doi: 10.1016/j.jhevol.2006.11.001
Shettleworth, S. J. (2010). Clever animals and killjoy explanations in comparative psychology. Trends Cogn. Sci. 14, 477–481. doi: 10.1016/j.tics.2010.07.002
Sperber, D. (1996). Explaining Culture : A Naturalistic Approach . Oxford: Wiley-Blackwell.
Sperber, D. (2000a). “Introduction,” in Metarepresentations: A Multidisciplinary Perspective , ed. D. Sperber (Oxford: Oxford University Press), 3–13.
Sperber, D. (eds) (2000b). Metarepresentations: A Multidisciplinary Perspective . Oxford: Oxford University Press.
Sperber, D., and Hirschfeld, L. A. (2004). The cognitive foundations of cultural stability and diversity. Trends Cogn. Sci. 8, 40–46. doi: 10.1016/j.tics.2003.11.002
Taylor, A. H., Elliffe, D. M., Hunt, G. R., Emery, N. J., Clayton, N. S., and Gray, R. D. (2011). New caledonian crows learn the functional properties of novel tool types. PLoS ONE 6:e26887. doi: 10.1371/journal.pone.0026887
Thornton, A., and Raihani, N. J. (2010). Identifying teaching in wild animals. Learn. Behav. 38, 297–309. doi: 10.3758/LB.38.3.297
Tomasello, M. (1990). “Cultural transmission in the tool use and communicatory signaling of chimpanzees?,” in ”Language” and Intelligence in Monkeys and Apes: Comparative Developmental Perspectives , eds S. Parker and K. Gibson (Cambridge: Cambridge University Press), 274–311.
Tomasello, M. (2009). “The question of chimpanzee culture, plus postscript (Chimpanzee culture, 2009),” in The Question of Animal Culture , eds K. N. Laland and B. G. Galef (Cambridge, MA: Harvard University Press), 198–221.
Tomasello, M., Carpenter, M., Call, J., Behne, T., and Moll, H. (2005). Understanding and sharing intentions: the origins of cultural cognition. Behav. Brain Sci. 28, 675–735. doi: 10.1017/S0140525X05000129
Tomasello, M., Melis, A. P., Tennie, C., Wyman, E., and Herrmann, E. (2012). Two key steps in the evolution of Human cooperation: the interdependence hypothesis. Curr. Anthropol. 53, 673–692. doi: 10.1086/668207
Träuble, B., and Pauen, S. (2007). The role of functional information for infant categorization. Cognition 105, 362–379. doi: 10.1016/j.cognition.2006.10.003
Vale, G. L., Flynn, E. G., and Kendal, R. L. (2012). Cumulative culture and future thinking: is mental time travel a prerequisite to cumulative cultural evolution? Learn. Motiv. 43, 220–230. doi: 10.1016/j.lmot.2012.05.010
van de Waal, E., Borgeaud, C., and Whiten, A. (2013). Potent social learning and conformity shape a wild Primate’s foraging decisions. Science 340, 483–485. doi: 10.1126/science.1232769
van de Waal, E., Renevey, N., Favre, C. M., and Bshary, R. (2010). Selective attention to philopatric models causes directed social learning in wild vervet monkeys. Proc. R. Soc. B Biol. Sci. 277, 2105–2111. doi: 10.1098/rspb.2009.2260
van Leeuwen, E. J. C., and Haun, D. B. M. (2013). Conformity in nonhuman primates: fad or fact? Evol. Hum. Behav. 34, 1–7. doi: 10.1016/j.evolhumbehav.2012.07.005
van Leeuwen, E. J. C., and Haun, D. B. M. (2014). Conformity without majority? The case for demarcating social from majority influences. Anim. Behav. 96, 187–194. doi: 10.1016/j.anbehav.2014.08.004
van Schaik, C. P. (2012). Animal culture: chimpanzee conformity? Curr. Biol. 22, R402–R404. doi: 10.1016/j.cub.2012.04.001
van Schaik, C. P., Ancrenaz, M., Borgen, G., Galdikas, B., Knott, C. D., Singleton, I.,et al. (2003). Orangutan cultures and the evolution of material culture. Science 299, 102–105. doi: 10.1126/science.1078004
van Schaik, C. P., Damerius, L., and Isler, K. (2013). Wild orangutan males plan and communicate their travel direction one day in advance. PLoS ONE 8:e74896. doi: 10.1371/journal.pone.0074896
Visalberghi, E., Adessi, E., Truppa, V., Spagnoletti, N., Ottoni, E., Izar, P.,et al. (2009). Selection of effective stone tools by wild bearded capuchin monkeys. Curr. Biol. 19, 213–217. doi: 10.1016/j.cub.2008.11.064
Warner, R. R. (1988). Traditionality of mating-site preferences in a coral reef fish. Nature 335, 719–721. doi: 10.1038/335719a0
Whiten, A. (2000). “Chimpanzee cognition and the question of mental re-representation,” in Metarepresentations: A Multidisciplinary Perspective , ed. D. Sperber (Oxford: Oxford University Press), 139–167.
Whiten, A., Goodall, J., Mcgrew, W. C., Nishida, T., Reynolds, V., Sugiyama, Y.,et al. (1999). Cultures in chimpanzees. Nature 399, 682–685. doi: 10.1038/21415
Whiten, A., Goodall, J., Mcgrew, W. C., Nishida, T., Reynolds, V., Sugiyama, Y.,et al. (2001). Charting cultural variation in chimpanzees. Behaviour 138, 1481–1516. doi: 10.1163/156853901317367717
Whiten, A., Horner, V., and De Waal, F. B. M. (2005). Conformity to cultural norms of tool use in chimpanzees. Nature 437, 737–740. doi: 10.1038/nature04047
Whiten, A., Mcguigan, N., Marshall-Pescini, S., and Hopper, L. M. (2009). Emulation, imitation, over-imitation and the scope of culture for child and chimpanzee. Philos. Trans. R. Soc. B Biol. Sci. 364, 2417–2428. doi: 10.1098/rstb.2009.0069
Whiten, A., Spiteri, A., Horner, V., Bonnie, K. E., Lambeth, S. P., Schapiro, S. J.,et al. (2007). Transmission of multiple traditions within and between chimpanzee groups. Curr. Biol. 17, 1038–1043. doi: 10.1016/j.cub.2007.05.031
Whiten, A., and van Schaik, C. P. (2007). The evolution of animal ‘cultures’ and social intelligence. Philos. Trans. R. Soc. B Biol. Sci. 362, 603–620. doi: 10.1098/rstb.2006.1998
Wilson, M. L., Boesch, C., Fruth, B., Furuichi, T., Gilby, I. C., Hashimoto, C.,et al. (2014). Lethal aggression in Pan is better explained by adaptive strategies than human impacts. Nature 513, 414–417. doi: 10.1038/nature13727
Yamamoto, S., Humle, T., and Tanaka, M. (2013). Basis for cumulative cultural evolution in chimpanzees: social learning of a more efficient tool-use technique. PLoS ONE 8:e55768. doi: 10.1371/journal.pone.0055768
Zuberbühler, K., Gygax, L., Harley, N., and Kummer, H. (1996). Stimulus enhancement and spread of a spontaneous tool use in a colony of long-tailed macaques. Primates 37, 1–12. doi: 10.1007/BF02382915
Keywords : animal culture, comparative cognition, field experiments, cultural mind, metarepresentation
Citation: Gruber T, Zuberbühler K, Clément F and van Schaik C (2015) Apes have culture but may not know that they do. Front. Psychol. 6 :91. doi: 10.3389/fpsyg.2015.00091
Received: 03 June 2014; Accepted: 16 January 2015; Published online: 06 February 2015.
Reviewed by:
Copyright © 2015 Gruber, Zuberbühler, Clément and van Schaik. This is an open-access article distributed under the terms of the Creative Commons Attribution License (CC BY) . The use, distribution or reproduction in other forums is permitted, provided the original author(s) or licensor are credited and that the original publication in this journal is cited, in accordance with accepted academic practice. No use, distribution or reproduction is permitted which does not comply with these terms.
*Correspondence: Thibaud Gruber, Department of Comparative Cognition, Institute of Biology, University of Neuchâtel, Rue Emile-Argand 11, Neuchâtel CH-2000, Switzerland e-mail: [email protected]
Disclaimer: All claims expressed in this article are solely those of the authors and do not necessarily represent those of their affiliated organizations, or those of the publisher, the editors and the reviewers. Any product that may be evaluated in this article or claim that may be made by its manufacturer is not guaranteed or endorsed by the publisher.
- Skip to main content
- Skip to primary sidebar
Anthropology
IResearchNet
Custom Writing Services
Aquatic ape hypothesis.
In 1960, Sir Alister Hardy, a marine biologist knighted for his contribution to the fisheries industry, gave a talk at the British Sub-Aqua Club (a scuba-diving club) and a month later published an article in New Scientist on that talk, called “Was Man More Aquatic in the Past?” Although the idea caught people’s fancies and garnered some attention by the newspapers, it showed little sign of the long-lasting popular appeal it would eventually have.
It didn’t happen overnight. Even with the original article and a follow-up transcript from a radio program, it was 7 years before Hardy’s idea got much notice—this time with a two-page write-up by Desmond Morris in The Naked Ape.
Elaine Morgan, at the time an Oxford graduate in English and a TV scriptwriter, entered the scene in 1972 with the book Descent of Women, the idea for which she got from Desmond Morris’s book. This book proved to be very popular, and in time Morgan followed it up with many articles as well as four more books on the subject (in 1982,1990,1994, and 1997).
During the 1980s, other proponents arrived on the scene, chief among them a medical doctor from Belgium, Marc Verhaegen. A 1987 conference on the subject resulted in a book presenting opposing views, The Aquatic Ape: Fact or Fiction?
The coming of age of the Internet in the 1990s brought the advent of the online venue for presentation and debate, and this subject proved popular. As with many subjects connected with humans and especially human evolution, much of the debate is rancorous and ill-informed. There are nuggets of gold in the online debate; however, just as with the mineral, finding these nuggets requires a lot of panning.
The Aquatic Ape Theory or Aquatic Ape Hypothesis (aka AAT or AAH—this entry will refer to it as the AAT/H) hypothesizes that humans went through an aquatic or semiaquatic stage in our evolution, generally said to have occurred during the transition from the last common ancestor we shared with apes (LCA) to hominids (some, like Marc Verhaegen, claim it continued on through virtually the entire span of human evolution). It claims that certain features are seen in human anatomy and physiology that are only seen in humans and aquatic animals and that these constitute conclusive evidence that our ancestors went through an semiaquatic phase in our evolution. Relying heavily on the principle of convergent evolution, it says that life in an aquatic environment explains these features and that a transition from ape to hominid in a nonaquatic environment cannot. The principle of convergence is used to explain these features, and the idea is said to be more parsimonious than other hypotheses.
Their use of the idea of convergence is generally accurate, but generally, they use parsimony to mean that only one cause explains many features (a more accurate term might be “prime mover” or “umbrella hypothesis,” the latter being one that AAT/H critic John Langdon uses).
One problem with the idea is that most of the proponents have been rather vague about the degree of aquaticness to which they refer. One proponent, doctoral student Algis Kuliukas, has come up with an explanation apparently also now endorsed by Elaine Morgan, that water has acted as more of an agent of selection in human evolution than in the evolution of apes such that physical differences between the two may be at least partly explained as adaptations to more efficient movement through acquatic conditions. This definition is perhaps most notable for its vagueness, merely suggesting “more” water use by humans than by apes. Contrast that with the explanation set forth in by Bininda-Emonds, Gittleman, and Kelly: “We consider aquatic carnivores to be those species in which the aquatic habitat inevitably plays a key role in the life-cycle of an individual,” which they then compare to definitions as given by others as they discuss the strengths and limitations of their own definition. The AAT/H definition suggested is not only so vague as to be virtually meaningless, it is notable that this is the first attempt at such an explicit definition, and it has taken over 40 years to show up. There has always been an implicit “how aquatic” definition, however, ascertained by the characteristics the proponents have used to build their case.
The proponents of the AAT/H have almost always been vague about just how aquatic our ancestors supposedly were, and now tend to say simply those ancestors were “more aquatic” than apes, and often use Alister Hardy’s suggestion that we were less aquatic than otters. Coupled with this, they are often coy about what animals are said to share our “aquatic” features, sometimes simply saying that these features are found in an aquatic setting or that we share these features with “aquatics.” This produces a disconnect between how aquatic most AAT/H proponents claim our ancestors were and what the idea clearly implies on this vital aspect.
The human features said to be accounted for by a semiaquatic past can vary a great deal from one AAT/H account to another, but they form a large and eclectic list, far too large to deal with here (various proponents have suggested sweat, tears, sebaceous glands, the shape of our nose and our thorax, and even being able to cup our hands better than chimps). Probably the most commonly cited features are bipedalism, fat characteristics, and the distribution of human hair. AAT/H proponents concede that bipedalism is not found in any aquatic or semiaquatic mammal. They use a double standard here, as they commonly argue that the idea that hominids evolved bipedality in a terrestrial setting is badly damaged by the fact that no nonhuman terrestrial mammal is predominantly bipedal, yet the fact that no aquatic mammal is bipedal is brushed off as irrelevant.
The mammals with the fat features the AAT/H proponents say are similar to ours are seals, whales, and the sirenia (dugongs and manatees) and for lessened hair, only whales and sirenia and one species of wild pig, the babirusa, which has less hair than other wild pigs and is at home in water but also seems to be, like humans, an exception, since other wild pigs that are at home in the water have plenty of body hair; the babirusa seems to be, like other mammals with lessened body hair (such as some mole rats), an exception among related mammals with similar habits. The pachyderms also have little body hair, and although this is generally accepted as being due to their size and the need for heat loss, this well-established physiological principle concerning volume versus surface area is viewed with suspicion by many AAT/H proponents as they concentrate on water as a guiding factor in evolution. So, the aquatic mammals the proponents say we resemble have all been aquatic for tens of millions of years and are so highly specialized they are virtually, or completely, incapable of living a non-aquatic life. The AAT/H claim that these features arose in a mammal less aquatic than an otter rings hollow.
So, relative fattiness and body hair loss among aquatic species are actually restricted to only a very few highly specialized aquatic mammals. Even more damaging to the AAT/H is that these features in aquatic animals do not actually resemble those of humans in any but the most superficial manner. This has particular significance in light of the AAT/H claim to be more parsimonious than other theories.
Most humans are fatter than many wild mammals, and we have, on average, less body hair than apes and most other wild mammals. But the characteristics of human hair and fat—the differences between the sexes and during the individual’s lifespan—are not like hair and fat features due to convergent evolution due to environment. Instead, those characteristics in humans seem to be classic cases of sexual selection. They differ considerably between the sexes, change dramatically at puberty, and then change again at the end of the reproductive period of life. (We are also unusual in having rather fat babies, but that seems to be connected to the fact that our infancy and accompanying brain growth is itself a highly unusual affair among primates, or any other mammal.) In aquatic mammals, these fat and hair characteristics either start out similar to adults of their species or become like adults of their species very rapidly after birth. This is also true of other features that AAT/H proponents use or have used as evidence, such as sweat and sebaceous glands.
The problem vis-à-vis parsimony is that if these were due to convergence during a semiaquatic past, the AAT/H requires two major changes to these features instead of one: first, ancestral, then similar to aquatic mammals, then sexually selected as in modern humans. Other hypotheses would require only one change, perhaps gradual: first ancestral, then sexually selected, albeit perhaps with some supporting advantages in natural selection (such as Wheeler’s cooling hypothesis or the recent idea that elimination of some parasites had a hand in our present hair characteristics). So, the AAT/H claim to be a more parsimonious explanation for these features falls apart.
Explanations for these discrepancies have been offered. Sometimes proponents actually suggest that the degree of aquaticness in hominids varied between the sexes and during the lifespan, so as to match the characteristics we see today. Since both sebaceous glands and hair are used as evidence, the hypothesis becomes internally inconsistent, since then females would have to be more aquatic than males to explain hair and body fat, while males would have to be more aquatic than females to explain sebaceous glands; babies would be aquatic to explain hair and body fat, but not very aquatic to explain sweat and sebaceous glands, while these same characteristics in children would mean they were relatively nonaquatic until puberty. These ad hoc explanations seem much more unlikely than the simple explanation of sexual selection.
There are other problems with AAT/H attempts to explain human hair as the result of some degree of aquaticness. The only reasonable suggestion as to why body hair would be reduced is to aid in swimming speed (some take the tack that it just is similar to aquatic mammals and offer no reason for the similarity— however, as mentioned already, it is not really similar). There is, however, conflicting evidence on body hair and swimming speed; it would seem that increasing body hair might work as well as eliminating it, but humans took neither course. We still have body hair, and many humans have quite a lot of it, often curly and just what we don’t want for increased swimming speed. That is why competitive swimmers use one or both of two methods—they shave off body hair and recently many have adopted special body suits that increase boundary layer thickness and decrease drag by mimicking the effects of dermal ridges as seen in dolphins’ skin or the hair of seals. The one thing competitive swimmers don’t want is what we have now, yet what we have now is what AAT/H proponents say was due to adaptation for swimming speed. And then there is the hair on our heads, which is far longer, and often bushier, than that of apes. So, the AAT/H proponents are left with several ad hoc explanations They have suggested that head hair is explained by a swimming stroke, usually said to be the breast stroke, which leaves the long head hair (and beard in males) entirely out of the water—and this unlikely position is supposed to be one which allows for high swimming speeds creating selection for hair loss. Otherwise, they are faced with claiming that there was intense environmental selective pressure for reduction of body hair but not for head hair. The only other explanation would be that there were two major changes to hair instead of one as required for nonaquatic hypotheses, which destroys their claim of parsimony.
The problem of likely aquatic predators such as crocodiles and sharks being much faster swimmers than even the best human athletes today is met with the suggestion that we congregated in large groups and the aquatic predators would only catch the slower members. This is unlikely to have helped even if true, since aquatic predators are seldom seen before they strike and would not have to take the slowest swimmers even if the herding idea was true. Still, that is better than Morgan’s first attempt to answer the problem of crocodiles, which was to label them “hypothetical.” No credible suggestion of how these ancestors would meet the problem of aquatic predators has ever been produced. Another problem for the hypothesis is the fact that while terrestrially we can show how an animal of medium size with a low birthrate can, and still does, deal with predators, by studying chimpanzees, no aquatic or semiaquatic animal of medium size with a low birthrate exists.
A common argument for the AAT/H over the years has been the fact that many humans like to visit the seashore, and many humans like to swim for recreation. This race memory argument has been used by both Hardy and Morgan (Hardy in particular believed in the race memory idea as part of his long-held belief that race memories and telepathy had played a role in human evolution) and carries no more weight, and perhaps less, than Gordon Orians’s idea that our liking for certain park settings is based on our evolutionary past on mixed savannas. Lately, the subject of fatty acids for brain growth, specifically DHA and LNA, have been promoted as support for the idea of an aquatic past, since marine fish, especially cold-water marine fish, are rich in DHA. This ignores the fact that these fatty acids are readily available in various plant sources as well as in wild game. Humans (except infants) can synthesize DHA from LNA found in plant foods; infants get it through breast milk.
Some evidence long used as support for the AAT/H has been dropped by its proponents, such as Morgan’s contention that sweat and tears were analogous to salt excretion glands, as seen in marine and desert reptiles and birds. The problem here is that even when this notion was first put forward, it ran counter to basic physiological principles in osmoregulation, including the fact that such excretions are never hypertonic, as they would have to be to be part of such a system. When Morgan formally dropped this line of evidence, she claimed it was because new information had been discovered that wasn’t available when she first proposed it, but in fact the contrary information was known well before she first brought it up, and some of that information was in the references she used as the sources for her claims. This does not speak well of the reliability of AAT/H research, and is not unusual.
Morgan has used a passage about bradycardia in seals, the slowing of the heart, as evidence about breath holding before dives. Morgan has also incorrectly stated that hymens are only found in humans and aquatic mammals; that humans, bonobos, and aquatic mammals are the only ones that engage in ventro-ven-tro copulation; that seals sweat via eccrine glands; that the only nonhuman animals that have been reported— none confirmed—to cry emotional tears are aquatic ones (information to the contrary was in the same book and chapter Morgan used as a source); and that the predominate mode of terrestrial locomotion of proboscis monkeys is bipedal. Morgan also has proved to exhibit something of a free hand when it comes to quotes in some of her AAT/H accounts, leaving out words or context to change their meaning, often with-out indicating the words are absent.
Marc Verhaegen has a history of dubious research. For example, he has claimed that sea lions are, after humans, the mammals that use sweat cooling the most, but in fact sea lions’ sweating mechanisms are not very efficient at all, unlike those of a variety of terrestrial mammals—that information was in sources that he referenced. Verhaegen has also suggested that Neandertal noses acted as snorkels and that ear canal exotoses, seen in a small number of Neandertal and erectus specimens, can only mean they were doing a great deal of swimming and diving. Problems with this are that such exotoses also result from exposure to cold air without swimming or diving, and it seems that a pathological condition would be weeded out in a species that was long adapted for swimming and diving. Other examples of the quality of his research include his description of the rhinoceros as “predominantly aquatic” and using the mountain beaver as an example of an aquatic mammal, apparently confused by the name (the mountain beaver often likes wet areas and burrows and succulent plants, but dampness does not make one aquatic in any realistic sense).
These are far from the only examples of poor AAT/H research, and unfortunately they typify the quality of research one sees when the idea is examined closely.
In recent years, proponents have paid a great deal of attention to the research on chimpanzees and gorillas that wade, along with any information about bonobos wading. This is an abrupt about-face, since as late as the mid-1990s, most were claiming as evidence the “fact” that common chimpanzees avoided water at all costs (a common misconception disproved well before that). The amount of bipedality in these situations is often wildly overstated by proponents, and another problem the AAT/H faces with these and other primate species that wade or swim regularly is that these species don’t exhibit the other changes the AAT/H predicts—changes such as fat and lessened body hair—but this problem is either downplayed or ignored. It is interesting that bonobos and other apes sometimes use bipedality when wading, and it is certainly one of the items on the list of things that apes sometimes use bipedality while doing, but the AAT/H attempts to be far more than one item on a list.
In summary, the AAT/H has been in existence for well over 40 years now, and while it has certainly attracted a following, it hasn’t been very convincing to most anthropologists who’ve looked at it closely. Its proponents tend to use to their advantage the fact that most people accept the proponents’ accounts of the features mentioned and assume that the proponents have been somewhat rigorous and honest in testing their theory against the evidence. And few people are conversant with all the varied lines of evidence that its proponents use. Perhaps a greater problem is that most people accept the proponents’ claim that they are doing no more than suggesting that humans used water somewhat more than apes during the evolution of our species—certainly a noncontroversial claim, but a disingenuous one as well when you see what features the AAT/H claims as evidence.
References:
- Bininda-Emonds, O. R. P., Gittleman, J. L., & Kelly, K. (2001). Flippers versus feet: Comparative trends in aquatic and non-aquatic carnivores. Journal of Animal Ecology, 70, 386-400.
- Morgan, E. (1990). The scars of evolution.London: Souvenir Press.
- Morgan, E. (1997). The aquatic ape hypothesis. London: Souvenir Press.
- Roede, M., Wind, J., Patrick, J. M., & Reynolds, V.(Eds.). (1991). The aquatic ape: Fact or fiction?London: Souvenir Press.

Reader Interactions
October 23, 2000
How closely related are humans to apes and other animals? How do scientists measure that? Are humans related to plants at all?
Bioinformatician Bernhard Haubold of the Max Planck Institute of Chemical Ecology provides this explanation:
Humans, chimpanzees, gorillas, orangutans and their extinct ancestors form a family of organisms known as the Hominidae. Researchers generally agree that among the living animals in this group, humans are most closely related to chimpanzees, judging from comparisons of anatomy and genetics.
On supporting science journalism
If you're enjoying this article, consider supporting our award-winning journalism by subscribing . By purchasing a subscription you are helping to ensure the future of impactful stories about the discoveries and ideas shaping our world today.
If life is the result of "descent with modification," as Charles Darwin put it, we can try to represent its history as a kind of family tree derived from these morphological and genetic characteristics. The tips of such a tree show organisms that are alive today. The nodes of the tree denote the common ancestors of all the tips connected to that node. Biologists refer to such nodes as the last common ancestor of a group of organisms, and all tips that connect to a particular node form a clade. In the diagram of the Hominidae at right, the clade designated by node 2 includes gorillas, humans and chimps. Within that clade the animal with which humans share the most recent common ancestor is the chimpanzee.
of the Hominidae shows that chimpanzees are our closest living relatives.
There are two major classes of evidence that allow us to estimate how old a particular clade is: fossil data and comparative data from living organisms. Fossils are conceptually easy to interpret. Once the age of the fossil is determined (using radiocarbon or thermoluminescence dating techniques, for example), we then know that an ancestor of the organism in question existed at least that long ago. There are, however, few good fossils available compared with the vast biodiversity around us. Thus, researchers also consider comparative data. We all know that siblings are more similar to each other than are cousins, which reflects the fact that siblings have a more recent common ancestor (parents) than do cousins (grandparents). Analogously, the greater similarity between humans and chimps than between humans and plants is taken as evidence that the last common ancestor of humans and chimps is far more recent than the last common ancestor of humans and plants. Similarity, in this context, refers to morphological features such as eyes and skeletal structure.
One problem with morphological data is that it is sometimes difficult to interpret. For example, ascertaining which similarities resulted from common ancestry and which resulted from convergent evolution can, on occasion, prove tricky. Furthermore, it is almost impossible to obtain time estimates from these data. So despite analyses of anatomy, the evolutionary relationships among many groups of organisms remained unclear due to lack of suitable data.
This changed in the 1950s and 1960s when protein sequence data and DNA sequence data, respectively, became available. The sequences of a protein (say, hemoglobin) from two organisms can be compared and the number of positions where the two sequences differ counted. It was soon learned from such studies that for a given protein, the number of amino acid substitutions per year could--as a first approximation--be treated as constant. This discovery became known as the "molecular clock." If the clock is calibrated using fossil data or data on continental drift, then the ages of various groups of organisms can theoretically be calculated based on comparisons of their sequences.
Using such reasoning, it has been estimated that the last common ancestor of humans and chimpanzees (with whom we share 99 percent of our genes) lived five million years ago. Going back a little farther, the Hominidae clade is 13 million years old. If we continue farther back in time, we find that placental mammals are between 60 and 80 million years old and that the oldest four-limbed animal, or tetrapod, lived between 300 and 350 million years ago and the earliest chordates (animals with a notochord) appeared about 990 million years ago. Humans belong to each of these successively broader groups.
How far back can we go in this way? If we try to trace all life on our planet, we are constrained by the earth's age of 4.5 billion years. The oldest bacteria-like fossils are 3.5 billion years old, so this is the upper estimate for the age of life on the earth. The question is whether at some point before this date a last common ancestor for all forms of life, a "universal ancestor," existed. Over the past 30 years the underlying biochemical unity of all plants, animals and microbes has become increasingly apparent. All organisms share a similar genetic machinery and certain biochemical motifs related to metabolism. It is therefore very likely that there once existed a universal ancestor and, in this sense, all things alive are related to each other. It took more than two billion years for this earliest form of life to evolve into the first eukaryotic cell. This gave rise to the last common ancestor of plants, fungi and animals, which lived some 1.6 billion years ago.
The controversies surrounding biological evolution today reflect the fact that biologists were late in accepting evolutionary thinking. One reason for this is that significant modifications of living things are difficult to observe during a lifetime. Darwin never saw evolution taking place in nature and had to rely on evidence from fossils, as well as plant and animal breeding. His idea that the differences observed within a species are transformed in time into differences between species remained the most plausible theory of biodiversity in his time, but there was an awkward lack of direct observations of this process. Today this situation has changed. There are now a number of very striking accounts of evolution in nature, including exceptional work on the finches of the Galapagos Islands--the same animals that first inspired Darwin's work.
FURTHER READING:
The Beak of the Finch : A Story of Evolution in Our Time (Vintage Books, 1995)

- Science Notes Posts
- Contact Science Notes
- Todd Helmenstine Biography
- Anne Helmenstine Biography
- Free Printable Periodic Tables (PDF and PNG)
- Periodic Table Wallpapers
- Interactive Periodic Table
- Periodic Table Posters
- Science Experiments for Kids
- How to Grow Crystals
- Chemistry Projects
- Fire and Flames Projects
- Holiday Science
- Chemistry Problems With Answers
- Physics Problems
- Unit Conversion Example Problems
- Chemistry Worksheets
- Biology Worksheets
- Periodic Table Worksheets
- Physical Science Worksheets
- Science Lab Worksheets
- My Amazon Books
Hypothesis Examples

A hypothesis is a prediction of the outcome of a test. It forms the basis for designing an experiment in the scientific method . A good hypothesis is testable, meaning it makes a prediction you can check with observation or experimentation. Here are different hypothesis examples.
Null Hypothesis Examples
The null hypothesis (H 0 ) is also known as the zero-difference or no-difference hypothesis. It predicts that changing one variable ( independent variable ) will have no effect on the variable being measured ( dependent variable ). Here are null hypothesis examples:
- Plant growth is unaffected by temperature.
- If you increase temperature, then solubility of salt will increase.
- Incidence of skin cancer is unrelated to ultraviolet light exposure.
- All brands of light bulb last equally long.
- Cats have no preference for the color of cat food.
- All daisies have the same number of petals.
Sometimes the null hypothesis shows there is a suspected correlation between two variables. For example, if you think plant growth is affected by temperature, you state the null hypothesis: “Plant growth is not affected by temperature.” Why do you do this, rather than say “If you change temperature, plant growth will be affected”? The answer is because it’s easier applying a statistical test that shows, with a high level of confidence, a null hypothesis is correct or incorrect.
Research Hypothesis Examples
A research hypothesis (H 1 ) is a type of hypothesis used to design an experiment. This type of hypothesis is often written as an if-then statement because it’s easy identifying the independent and dependent variables and seeing how one affects the other. If-then statements explore cause and effect. In other cases, the hypothesis shows a correlation between two variables. Here are some research hypothesis examples:
- If you leave the lights on, then it takes longer for people to fall asleep.
- If you refrigerate apples, they last longer before going bad.
- If you keep the curtains closed, then you need less electricity to heat or cool the house (the electric bill is lower).
- If you leave a bucket of water uncovered, then it evaporates more quickly.
- Goldfish lose their color if they are not exposed to light.
- Workers who take vacations are more productive than those who never take time off.
Is It Okay to Disprove a Hypothesis?
Yes! You may even choose to write your hypothesis in such a way that it can be disproved because it’s easier to prove a statement is wrong than to prove it is right. In other cases, if your prediction is incorrect, that doesn’t mean the science is bad. Revising a hypothesis is common. It demonstrates you learned something you did not know before you conducted the experiment.
Test yourself with a Scientific Method Quiz .
- Mellenbergh, G.J. (2008). Chapter 8: Research designs: Testing of research hypotheses. In H.J. Adèr & G.J. Mellenbergh (eds.), Advising on Research Methods: A Consultant’s Companion . Huizen, The Netherlands: Johannes van Kessel Publishing.
- Popper, Karl R. (1959). The Logic of Scientific Discovery . Hutchinson & Co. ISBN 3-1614-8410-X.
- Schick, Theodore; Vaughn, Lewis (2002). How to think about weird things: critical thinking for a New Age . Boston: McGraw-Hill Higher Education. ISBN 0-7674-2048-9.
- Tobi, Hilde; Kampen, Jarl K. (2018). “Research design: the methodology for interdisciplinary research framework”. Quality & Quantity . 52 (3): 1209–1225. doi: 10.1007/s11135-017-0513-8
Related Posts

15 Hypothesis Examples
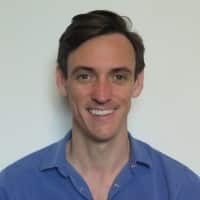
Chris Drew (PhD)
Dr. Chris Drew is the founder of the Helpful Professor. He holds a PhD in education and has published over 20 articles in scholarly journals. He is the former editor of the Journal of Learning Development in Higher Education. [Image Descriptor: Photo of Chris]
Learn about our Editorial Process
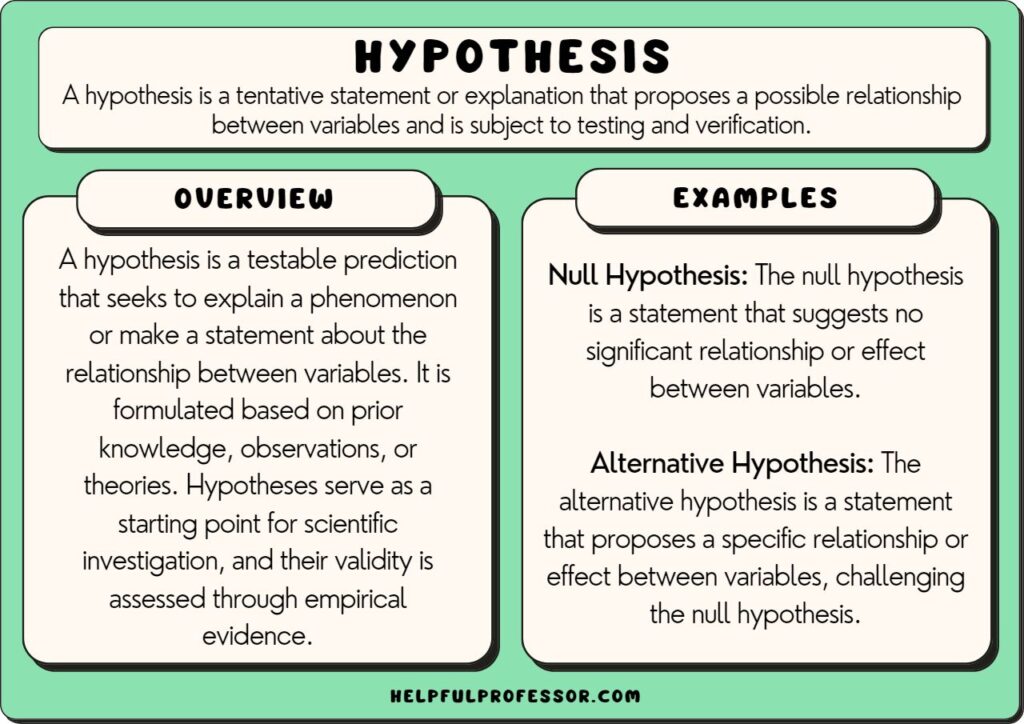
A hypothesis is defined as a testable prediction , and is used primarily in scientific experiments as a potential or predicted outcome that scientists attempt to prove or disprove (Atkinson et al., 2021; Tan, 2022).
In my types of hypothesis article, I outlined 13 different hypotheses, including the directional hypothesis (which makes a prediction about an effect of a treatment will be positive or negative) and the associative hypothesis (which makes a prediction about the association between two variables).
This article will dive into some interesting examples of hypotheses and examine potential ways you might test each one.
Hypothesis Examples
1. “inadequate sleep decreases memory retention”.
Field: Psychology
Type: Causal Hypothesis A causal hypothesis explores the effect of one variable on another. This example posits that a lack of adequate sleep causes decreased memory retention. In other words, if you are not getting enough sleep, your ability to remember and recall information may suffer.
How to Test:
To test this hypothesis, you might devise an experiment whereby your participants are divided into two groups: one receives an average of 8 hours of sleep per night for a week, while the other gets less than the recommended sleep amount.
During this time, all participants would daily study and recall new, specific information. You’d then measure memory retention of this information for both groups using standard memory tests and compare the results.
Should the group with less sleep have statistically significant poorer memory scores, the hypothesis would be supported.
Ensuring the integrity of the experiment requires taking into account factors such as individual health differences, stress levels, and daily nutrition.
Relevant Study: Sleep loss, learning capacity and academic performance (Curcio, Ferrara & De Gennaro, 2006)
2. “Increase in Temperature Leads to Increase in Kinetic Energy”
Field: Physics
Type: Deductive Hypothesis The deductive hypothesis applies the logic of deductive reasoning – it moves from a general premise to a more specific conclusion. This specific hypothesis assumes that as temperature increases, the kinetic energy of particles also increases – that is, when you heat something up, its particles move around more rapidly.
This hypothesis could be examined by heating a gas in a controlled environment and capturing the movement of its particles as a function of temperature.
You’d gradually increase the temperature and measure the kinetic energy of the gas particles with each increment. If the kinetic energy consistently rises with the temperature, your hypothesis gets supporting evidence.
Variables such as pressure and volume of the gas would need to be held constant to ensure validity of results.
3. “Children Raised in Bilingual Homes Develop Better Cognitive Skills”
Field: Psychology/Linguistics
Type: Comparative Hypothesis The comparative hypothesis posits a difference between two or more groups based on certain variables. In this context, you might propose that children raised in bilingual homes have superior cognitive skills compared to those raised in monolingual homes.
Testing this hypothesis could involve identifying two groups of children: those raised in bilingual homes, and those raised in monolingual homes.
Cognitive skills in both groups would be evaluated using a standard cognitive ability test at different stages of development. The examination would be repeated over a significant time period for consistency.
If the group raised in bilingual homes persistently scores higher than the other, the hypothesis would thereby be supported.
The challenge for the researcher would be controlling for other variables that could impact cognitive development, such as socio-economic status, education level of parents, and parenting styles.
Relevant Study: The cognitive benefits of being bilingual (Marian & Shook, 2012)
4. “High-Fiber Diet Leads to Lower Incidences of Cardiovascular Diseases”
Field: Medicine/Nutrition
Type: Alternative Hypothesis The alternative hypothesis suggests an alternative to a null hypothesis. In this context, the implied null hypothesis could be that diet has no effect on cardiovascular health, which the alternative hypothesis contradicts by suggesting that a high-fiber diet leads to fewer instances of cardiovascular diseases.
To test this hypothesis, a longitudinal study could be conducted on two groups of participants; one adheres to a high-fiber diet, while the other follows a diet low in fiber.
After a fixed period, the cardiovascular health of participants in both groups could be analyzed and compared. If the group following a high-fiber diet has a lower number of recorded cases of cardiovascular diseases, it would provide evidence supporting the hypothesis.
Control measures should be implemented to exclude the influence of other lifestyle and genetic factors that contribute to cardiovascular health.
Relevant Study: Dietary fiber, inflammation, and cardiovascular disease (King, 2005)
5. “Gravity Influences the Directional Growth of Plants”
Field: Agronomy / Botany
Type: Explanatory Hypothesis An explanatory hypothesis attempts to explain a phenomenon. In this case, the hypothesis proposes that gravity affects how plants direct their growth – both above-ground (toward sunlight) and below-ground (towards water and other resources).
The testing could be conducted by growing plants in a rotating cylinder to create artificial gravity.
Observations on the direction of growth, over a specified period, can provide insights into the influencing factors. If plants consistently direct their growth in a manner that indicates the influence of gravitational pull, the hypothesis is substantiated.
It is crucial to ensure that other growth-influencing factors, such as light and water, are uniformly distributed so that only gravity influences the directional growth.
6. “The Implementation of Gamified Learning Improves Students’ Motivation”
Field: Education
Type: Relational Hypothesis The relational hypothesis describes the relation between two variables. Here, the hypothesis is that the implementation of gamified learning has a positive effect on the motivation of students.
To validate this proposition, two sets of classes could be compared: one that implements a learning approach with game-based elements, and another that follows a traditional learning approach.
The students’ motivation levels could be gauged by monitoring their engagement, performance, and feedback over a considerable timeframe.
If the students engaged in the gamified learning context present higher levels of motivation and achievement, the hypothesis would be supported.
Control measures ought to be put into place to account for individual differences, including prior knowledge and attitudes towards learning.
Relevant Study: Does educational gamification improve students’ motivation? (Chapman & Rich, 2018)
7. “Mathematics Anxiety Negatively Affects Performance”
Field: Educational Psychology
Type: Research Hypothesis The research hypothesis involves making a prediction that will be tested. In this case, the hypothesis proposes that a student’s anxiety about math can negatively influence their performance in math-related tasks.
To assess this hypothesis, researchers must first measure the mathematics anxiety levels of a sample of students using a validated instrument, such as the Mathematics Anxiety Rating Scale.
Then, the students’ performance in mathematics would be evaluated through standard testing. If there’s a negative correlation between the levels of math anxiety and math performance (meaning as anxiety increases, performance decreases), the hypothesis would be supported.
It would be crucial to control for relevant factors such as overall academic performance and previous mathematical achievement.
8. “Disruption of Natural Sleep Cycle Impairs Worker Productivity”
Field: Organizational Psychology
Type: Operational Hypothesis The operational hypothesis involves defining the variables in measurable terms. In this example, the hypothesis posits that disrupting the natural sleep cycle, for instance through shift work or irregular working hours, can lessen productivity among workers.
To test this hypothesis, you could collect data from workers who maintain regular working hours and those with irregular schedules.
Measuring productivity could involve examining the worker’s ability to complete tasks, the quality of their work, and their efficiency.
If workers with interrupted sleep cycles demonstrate lower productivity compared to those with regular sleep patterns, it would lend support to the hypothesis.
Consideration should be given to potential confounding variables such as job type, worker age, and overall health.
9. “Regular Physical Activity Reduces the Risk of Depression”
Field: Health Psychology
Type: Predictive Hypothesis A predictive hypothesis involves making a prediction about the outcome of a study based on the observed relationship between variables. In this case, it is hypothesized that individuals who engage in regular physical activity are less likely to suffer from depression.
Longitudinal studies would suit to test this hypothesis, tracking participants’ levels of physical activity and their mental health status over time.
The level of physical activity could be self-reported or monitored, while mental health status could be assessed using standard diagnostic tools or surveys.
If data analysis shows that participants maintaining regular physical activity have a lower incidence of depression, this would endorse the hypothesis.
However, care should be taken to control other lifestyle and behavioral factors that could intervene with the results.
Relevant Study: Regular physical exercise and its association with depression (Kim, 2022)
10. “Regular Meditation Enhances Emotional Stability”
Type: Empirical Hypothesis In the empirical hypothesis, predictions are based on amassed empirical evidence . This particular hypothesis theorizes that frequent meditation leads to improved emotional stability, resonating with numerous studies linking meditation to a variety of psychological benefits.
Earlier studies reported some correlations, but to test this hypothesis directly, you’d organize an experiment where one group meditates regularly over a set period while a control group doesn’t.
Both groups’ emotional stability levels would be measured at the start and end of the experiment using a validated emotional stability assessment.
If regular meditators display noticeable improvements in emotional stability compared to the control group, the hypothesis gains credit.
You’d have to ensure a similar emotional baseline for all participants at the start to avoid skewed results.
11. “Children Exposed to Reading at an Early Age Show Superior Academic Progress”
Type: Directional Hypothesis The directional hypothesis predicts the direction of an expected relationship between variables. Here, the hypothesis anticipates that early exposure to reading positively affects a child’s academic advancement.
A longitudinal study tracking children’s reading habits from an early age and their consequent academic performance could validate this hypothesis.
Parents could report their children’s exposure to reading at home, while standardized school exam results would provide a measure of academic achievement.
If the children exposed to early reading consistently perform better acadically, it gives weight to the hypothesis.
However, it would be important to control for variables that might impact academic performance, such as socioeconomic background, parental education level, and school quality.
12. “Adopting Energy-efficient Technologies Reduces Carbon Footprint of Industries”
Field: Environmental Science
Type: Descriptive Hypothesis A descriptive hypothesis predicts the existence of an association or pattern related to variables. In this scenario, the hypothesis suggests that industries adopting energy-efficient technologies will resultantly show a reduced carbon footprint.
Global industries making use of energy-efficient technologies could track their carbon emissions over time. At the same time, others not implementing such technologies continue their regular tracking.
After a defined time, the carbon emission data of both groups could be compared. If industries that adopted energy-efficient technologies demonstrate a notable reduction in their carbon footprints, the hypothesis would hold strong.
In the experiment, you would exclude variations brought by factors such as industry type, size, and location.
13. “Reduced Screen Time Improves Sleep Quality”
Type: Simple Hypothesis The simple hypothesis is a prediction about the relationship between two variables, excluding any other variables from consideration. This example posits that by reducing time spent on devices like smartphones and computers, an individual should experience improved sleep quality.
A sample group would need to reduce their daily screen time for a pre-determined period. Sleep quality before and after the reduction could be measured using self-report sleep diaries and objective measures like actigraphy, monitoring movement and wakefulness during sleep.
If the data shows that sleep quality improved post the screen time reduction, the hypothesis would be validated.
Other aspects affecting sleep quality, like caffeine intake, should be controlled during the experiment.
Relevant Study: Screen time use impacts low‐income preschool children’s sleep quality, tiredness, and ability to fall asleep (Waller et al., 2021)
14. Engaging in Brain-Training Games Improves Cognitive Functioning in Elderly
Field: Gerontology
Type: Inductive Hypothesis Inductive hypotheses are based on observations leading to broader generalizations and theories. In this context, the hypothesis deduces from observed instances that engaging in brain-training games can help improve cognitive functioning in the elderly.
A longitudinal study could be conducted where an experimental group of elderly people partakes in regular brain-training games.
Their cognitive functioning could be assessed at the start of the study and at regular intervals using standard neuropsychological tests.
If the group engaging in brain-training games shows better cognitive functioning scores over time compared to a control group not playing these games, the hypothesis would be supported.
15. Farming Practices Influence Soil Erosion Rates
Type: Null Hypothesis A null hypothesis is a negative statement assuming no relationship or difference between variables. The hypothesis in this context asserts there’s no effect of different farming practices on the rates of soil erosion.
Comparing soil erosion rates in areas with different farming practices over a considerable timeframe could help test this hypothesis.
If, statistically, the farming practices do not lead to differences in soil erosion rates, the null hypothesis is accepted.
However, if marked variation appears, the null hypothesis is rejected, meaning farming practices do influence soil erosion rates. It would be crucial to control for external factors like weather, soil type, and natural vegetation.
The variety of hypotheses mentioned above underscores the diversity of research constructs inherent in different fields, each with its unique purpose and way of testing.
While researchers may develop hypotheses primarily as tools to define and narrow the focus of the study, these hypotheses also serve as valuable guiding forces for the data collection and analysis procedures, making the research process more efficient and direction-focused.
Hypotheses serve as a compass for any form of academic research. The diverse examples provided, from Psychology to Educational Studies, Environmental Science to Gerontology, clearly demonstrate how certain hypotheses suit specific fields more aptly than others.
It is important to underline that although these varied hypotheses differ in their structure and methods of testing, each endorses the fundamental value of empiricism in research. Evidence-based decision making remains at the heart of scholarly inquiry, regardless of the research field, thus aligning all hypotheses to the core purpose of scientific investigation.
Testing hypotheses is an essential part of the scientific method . By doing so, researchers can either confirm their predictions, giving further validity to an existing theory, or they might uncover new insights that could potentially shift the field’s understanding of a particular phenomenon. In either case, hypotheses serve as the stepping stones for scientific exploration and discovery.
Atkinson, P., Delamont, S., Cernat, A., Sakshaug, J. W., & Williams, R. A. (2021). SAGE research methods foundations . SAGE Publications Ltd.
Curcio, G., Ferrara, M., & De Gennaro, L. (2006). Sleep loss, learning capacity and academic performance. Sleep medicine reviews , 10 (5), 323-337.
Kim, J. H. (2022). Regular physical exercise and its association with depression: A population-based study short title: Exercise and depression. Psychiatry Research , 309 , 114406.
King, D. E. (2005). Dietary fiber, inflammation, and cardiovascular disease. Molecular nutrition & food research , 49 (6), 594-600.
Marian, V., & Shook, A. (2012, September). The cognitive benefits of being bilingual. In Cerebrum: the Dana forum on brain science (Vol. 2012). Dana Foundation.
Tan, W. C. K. (2022). Research Methods: A Practical Guide For Students And Researchers (Second Edition) . World Scientific Publishing Company.
Waller, N. A., Zhang, N., Cocci, A. H., D’Agostino, C., Wesolek‐Greenson, S., Wheelock, K., … & Resnicow, K. (2021). Screen time use impacts low‐income preschool children’s sleep quality, tiredness, and ability to fall asleep. Child: care, health and development, 47 (5), 618-626.
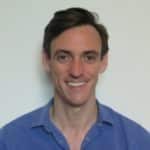
- Chris Drew (PhD) https://helpfulprofessor.com/author/chris-drew-phd-2/ 10 Reasons you’re Perpetually Single
- Chris Drew (PhD) https://helpfulprofessor.com/author/chris-drew-phd-2/ 20 Montessori Toddler Bedrooms (Design Inspiration)
- Chris Drew (PhD) https://helpfulprofessor.com/author/chris-drew-phd-2/ 21 Montessori Homeschool Setups
- Chris Drew (PhD) https://helpfulprofessor.com/author/chris-drew-phd-2/ 101 Hidden Talents Examples
Leave a Comment Cancel Reply
Your email address will not be published. Required fields are marked *
- Bipolar Disorder
- Therapy Center
- When To See a Therapist
- Types of Therapy
- Best Online Therapy
- Best Couples Therapy
- Managing Stress
- Sleep and Dreaming
- Understanding Emotions
- Self-Improvement
- Healthy Relationships
- Student Resources
- Personality Types
- Sweepstakes
- Guided Meditations
- Verywell Mind Insights
- 2024 Verywell Mind 25
- Mental Health in the Classroom
- Editorial Process
- Meet Our Review Board
- Crisis Support
How to Write a Great Hypothesis
Hypothesis Definition, Format, Examples, and Tips
Verywell / Alex Dos Diaz
- The Scientific Method
Hypothesis Format
Falsifiability of a hypothesis.
- Operationalization
Hypothesis Types
Hypotheses examples.
- Collecting Data
A hypothesis is a tentative statement about the relationship between two or more variables. It is a specific, testable prediction about what you expect to happen in a study. It is a preliminary answer to your question that helps guide the research process.
Consider a study designed to examine the relationship between sleep deprivation and test performance. The hypothesis might be: "This study is designed to assess the hypothesis that sleep-deprived people will perform worse on a test than individuals who are not sleep-deprived."
At a Glance
A hypothesis is crucial to scientific research because it offers a clear direction for what the researchers are looking to find. This allows them to design experiments to test their predictions and add to our scientific knowledge about the world. This article explores how a hypothesis is used in psychology research, how to write a good hypothesis, and the different types of hypotheses you might use.
The Hypothesis in the Scientific Method
In the scientific method , whether it involves research in psychology, biology, or some other area, a hypothesis represents what the researchers think will happen in an experiment. The scientific method involves the following steps:
- Forming a question
- Performing background research
- Creating a hypothesis
- Designing an experiment
- Collecting data
- Analyzing the results
- Drawing conclusions
- Communicating the results
The hypothesis is a prediction, but it involves more than a guess. Most of the time, the hypothesis begins with a question which is then explored through background research. At this point, researchers then begin to develop a testable hypothesis.
Unless you are creating an exploratory study, your hypothesis should always explain what you expect to happen.
In a study exploring the effects of a particular drug, the hypothesis might be that researchers expect the drug to have some type of effect on the symptoms of a specific illness. In psychology, the hypothesis might focus on how a certain aspect of the environment might influence a particular behavior.
Remember, a hypothesis does not have to be correct. While the hypothesis predicts what the researchers expect to see, the goal of the research is to determine whether this guess is right or wrong. When conducting an experiment, researchers might explore numerous factors to determine which ones might contribute to the ultimate outcome.
In many cases, researchers may find that the results of an experiment do not support the original hypothesis. When writing up these results, the researchers might suggest other options that should be explored in future studies.
In many cases, researchers might draw a hypothesis from a specific theory or build on previous research. For example, prior research has shown that stress can impact the immune system. So a researcher might hypothesize: "People with high-stress levels will be more likely to contract a common cold after being exposed to the virus than people who have low-stress levels."
In other instances, researchers might look at commonly held beliefs or folk wisdom. "Birds of a feather flock together" is one example of folk adage that a psychologist might try to investigate. The researcher might pose a specific hypothesis that "People tend to select romantic partners who are similar to them in interests and educational level."
Elements of a Good Hypothesis
So how do you write a good hypothesis? When trying to come up with a hypothesis for your research or experiments, ask yourself the following questions:
- Is your hypothesis based on your research on a topic?
- Can your hypothesis be tested?
- Does your hypothesis include independent and dependent variables?
Before you come up with a specific hypothesis, spend some time doing background research. Once you have completed a literature review, start thinking about potential questions you still have. Pay attention to the discussion section in the journal articles you read . Many authors will suggest questions that still need to be explored.
How to Formulate a Good Hypothesis
To form a hypothesis, you should take these steps:
- Collect as many observations about a topic or problem as you can.
- Evaluate these observations and look for possible causes of the problem.
- Create a list of possible explanations that you might want to explore.
- After you have developed some possible hypotheses, think of ways that you could confirm or disprove each hypothesis through experimentation. This is known as falsifiability.
In the scientific method , falsifiability is an important part of any valid hypothesis. In order to test a claim scientifically, it must be possible that the claim could be proven false.
Students sometimes confuse the idea of falsifiability with the idea that it means that something is false, which is not the case. What falsifiability means is that if something was false, then it is possible to demonstrate that it is false.
One of the hallmarks of pseudoscience is that it makes claims that cannot be refuted or proven false.
The Importance of Operational Definitions
A variable is a factor or element that can be changed and manipulated in ways that are observable and measurable. However, the researcher must also define how the variable will be manipulated and measured in the study.
Operational definitions are specific definitions for all relevant factors in a study. This process helps make vague or ambiguous concepts detailed and measurable.
For example, a researcher might operationally define the variable " test anxiety " as the results of a self-report measure of anxiety experienced during an exam. A "study habits" variable might be defined by the amount of studying that actually occurs as measured by time.
These precise descriptions are important because many things can be measured in various ways. Clearly defining these variables and how they are measured helps ensure that other researchers can replicate your results.
Replicability
One of the basic principles of any type of scientific research is that the results must be replicable.
Replication means repeating an experiment in the same way to produce the same results. By clearly detailing the specifics of how the variables were measured and manipulated, other researchers can better understand the results and repeat the study if needed.
Some variables are more difficult than others to define. For example, how would you operationally define a variable such as aggression ? For obvious ethical reasons, researchers cannot create a situation in which a person behaves aggressively toward others.
To measure this variable, the researcher must devise a measurement that assesses aggressive behavior without harming others. The researcher might utilize a simulated task to measure aggressiveness in this situation.

Hypothesis Checklist
- Does your hypothesis focus on something that you can actually test?
- Does your hypothesis include both an independent and dependent variable?
- Can you manipulate the variables?
- Can your hypothesis be tested without violating ethical standards?
The hypothesis you use will depend on what you are investigating and hoping to find. Some of the main types of hypotheses that you might use include:
- Simple hypothesis : This type of hypothesis suggests there is a relationship between one independent variable and one dependent variable.
- Complex hypothesis : This type suggests a relationship between three or more variables, such as two independent and dependent variables.
- Null hypothesis : This hypothesis suggests no relationship exists between two or more variables.
- Alternative hypothesis : This hypothesis states the opposite of the null hypothesis.
- Statistical hypothesis : This hypothesis uses statistical analysis to evaluate a representative population sample and then generalizes the findings to the larger group.
- Logical hypothesis : This hypothesis assumes a relationship between variables without collecting data or evidence.
A hypothesis often follows a basic format of "If {this happens} then {this will happen}." One way to structure your hypothesis is to describe what will happen to the dependent variable if you change the independent variable .
The basic format might be: "If {these changes are made to a certain independent variable}, then we will observe {a change in a specific dependent variable}."
A few examples of simple hypotheses:
- "Students who eat breakfast will perform better on a math exam than students who do not eat breakfast."
- "Students who experience test anxiety before an English exam will get lower scores than students who do not experience test anxiety."
- "Motorists who talk on the phone while driving will be more likely to make errors on a driving course than those who do not talk on the phone."
- "Children who receive a new reading intervention will have higher reading scores than students who do not receive the intervention."
Examples of a complex hypothesis include:
- "People with high-sugar diets and sedentary activity levels are more likely to develop depression."
- "Younger people who are regularly exposed to green, outdoor areas have better subjective well-being than older adults who have limited exposure to green spaces."
Examples of a null hypothesis include:
- "There is no difference in anxiety levels between people who take St. John's wort supplements and those who do not."
- "There is no difference in scores on a memory recall task between children and adults."
- "There is no difference in aggression levels between children who play first-person shooter games and those who do not."
Examples of an alternative hypothesis:
- "People who take St. John's wort supplements will have less anxiety than those who do not."
- "Adults will perform better on a memory task than children."
- "Children who play first-person shooter games will show higher levels of aggression than children who do not."
Collecting Data on Your Hypothesis
Once a researcher has formed a testable hypothesis, the next step is to select a research design and start collecting data. The research method depends largely on exactly what they are studying. There are two basic types of research methods: descriptive research and experimental research.
Descriptive Research Methods
Descriptive research such as case studies , naturalistic observations , and surveys are often used when conducting an experiment is difficult or impossible. These methods are best used to describe different aspects of a behavior or psychological phenomenon.
Once a researcher has collected data using descriptive methods, a correlational study can examine how the variables are related. This research method might be used to investigate a hypothesis that is difficult to test experimentally.
Experimental Research Methods
Experimental methods are used to demonstrate causal relationships between variables. In an experiment, the researcher systematically manipulates a variable of interest (known as the independent variable) and measures the effect on another variable (known as the dependent variable).
Unlike correlational studies, which can only be used to determine if there is a relationship between two variables, experimental methods can be used to determine the actual nature of the relationship—whether changes in one variable actually cause another to change.
The hypothesis is a critical part of any scientific exploration. It represents what researchers expect to find in a study or experiment. In situations where the hypothesis is unsupported by the research, the research still has value. Such research helps us better understand how different aspects of the natural world relate to one another. It also helps us develop new hypotheses that can then be tested in the future.
Thompson WH, Skau S. On the scope of scientific hypotheses . R Soc Open Sci . 2023;10(8):230607. doi:10.1098/rsos.230607
Taran S, Adhikari NKJ, Fan E. Falsifiability in medicine: what clinicians can learn from Karl Popper [published correction appears in Intensive Care Med. 2021 Jun 17;:]. Intensive Care Med . 2021;47(9):1054-1056. doi:10.1007/s00134-021-06432-z
Eyler AA. Research Methods for Public Health . 1st ed. Springer Publishing Company; 2020. doi:10.1891/9780826182067.0004
Nosek BA, Errington TM. What is replication ? PLoS Biol . 2020;18(3):e3000691. doi:10.1371/journal.pbio.3000691
Aggarwal R, Ranganathan P. Study designs: Part 2 - Descriptive studies . Perspect Clin Res . 2019;10(1):34-36. doi:10.4103/picr.PICR_154_18
Nevid J. Psychology: Concepts and Applications. Wadworth, 2013.
By Kendra Cherry, MSEd Kendra Cherry, MS, is a psychosocial rehabilitation specialist, psychology educator, and author of the "Everything Psychology Book."
What Are Examples of a Hypothesis?
- Scientific Method
- Chemical Laws
- Periodic Table
- Projects & Experiments
- Biochemistry
- Physical Chemistry
- Medical Chemistry
- Chemistry In Everyday Life
- Famous Chemists
- Activities for Kids
- Abbreviations & Acronyms
- Weather & Climate
- Ph.D., Biomedical Sciences, University of Tennessee at Knoxville
- B.A., Physics and Mathematics, Hastings College
A hypothesis is an explanation for a set of observations. Hypothesis examples can help you understand how this scientific method works.
Although you could state a scientific hypothesis in various ways, most hypotheses are either "If, then" statements or forms of the null hypothesis. The null hypothesis is sometimes called the "no difference" hypothesis. The null hypothesis is good for experimentation because it's simple to disprove. If you disprove a null hypothesis, that is evidence for a relationship between the variables you are examining.
Hypotheses Examples: Null
- All daisies have the same number of petals.
- Hyperactivity is unrelated to eating sugar.
- The number of pets in a household is unrelated to the number of people living in it.
- A person's preference for a shirt is unrelated to its color.
Hypotheses Examples: If, Then
- If you get at least 6 hours of sleep, you will do better on tests than if you get less sleep.
- If you drop a ball, it will fall toward the ground.
- If you drink coffee before going to bed, then it will take longer to fall asleep.
- If you cover a wound with a bandage, then it will heal with less scarring.
Improving a Hypothesis to Make It Testable
You may wish to revise your first hypothesis to make it easier to design an experiment to test. For example, let's say you have a bad breakout the morning after eating a lot of greasy food. You may wonder if there is a correlation between eating greasy food and getting pimples. You propose the hypothesis example:
Eating greasy food causes pimples.
Next, you need to design an experiment to test this hypothesis. Let's say you decide to eat greasy food every day for a week and record the effect on your face. Then, as a control, you'll avoid greasy food for the next week and see what happens. Now, this is not a good experiment because it does not take into account other factors such as hormone levels, stress, sun exposure, exercise, or any number of other variables that might conceivably affect your skin.
The problem is that you cannot assign cause to your effect . If you eat french fries for a week and suffer a breakout, can you definitely say it was the grease in the food that caused it? Maybe it was the salt. Maybe it was the potato. Maybe it was unrelated to diet. You can't prove your hypothesis. It's much easier to disprove a hypothesis.
So, let's restate the hypothesis to make it easier to evaluate the data:
Getting pimples is unaffected by eating greasy food.
So, if you eat fatty food every day for a week and suffer breakouts and then don't break out the week that you avoid greasy food, you can be pretty sure something is up. Can you disprove the hypothesis? Probably not, since it is so hard to assign cause and effect. However, you can make a strong case that there is some relationship between diet and acne.
If your skin stays clear for the entire test, you may decide to accept your hypothesis . Again, you didn't prove or disprove anything, which is fine
- Null Hypothesis Examples
- The Role of a Controlled Variable in an Experiment
- Random Error vs. Systematic Error
- What Is a Testable Hypothesis?
- What Are the Elements of a Good Hypothesis?
- Scientific Hypothesis Examples
- What Is a Hypothesis? (Science)
- Scientific Method Vocabulary Terms
- Scientific Method Flow Chart
- Understanding Simple vs Controlled Experiments
- Six Steps of the Scientific Method
- What Is an Experimental Constant?
- What Is the Difference Between a Control Variable and Control Group?
- Scientific Variable
- What Is a Controlled Experiment?
- DRY MIX Experiment Variables Acronym
Have a language expert improve your writing
Run a free plagiarism check in 10 minutes, generate accurate citations for free.
- Knowledge Base
Methodology
- How to Write a Strong Hypothesis | Steps & Examples
How to Write a Strong Hypothesis | Steps & Examples
Published on May 6, 2022 by Shona McCombes . Revised on November 20, 2023.
A hypothesis is a statement that can be tested by scientific research. If you want to test a relationship between two or more variables, you need to write hypotheses before you start your experiment or data collection .
Example: Hypothesis
Daily apple consumption leads to fewer doctor’s visits.
Table of contents
What is a hypothesis, developing a hypothesis (with example), hypothesis examples, other interesting articles, frequently asked questions about writing hypotheses.
A hypothesis states your predictions about what your research will find. It is a tentative answer to your research question that has not yet been tested. For some research projects, you might have to write several hypotheses that address different aspects of your research question.
A hypothesis is not just a guess – it should be based on existing theories and knowledge. It also has to be testable, which means you can support or refute it through scientific research methods (such as experiments, observations and statistical analysis of data).
Variables in hypotheses
Hypotheses propose a relationship between two or more types of variables .
- An independent variable is something the researcher changes or controls.
- A dependent variable is something the researcher observes and measures.
If there are any control variables , extraneous variables , or confounding variables , be sure to jot those down as you go to minimize the chances that research bias will affect your results.
In this example, the independent variable is exposure to the sun – the assumed cause . The dependent variable is the level of happiness – the assumed effect .
Here's why students love Scribbr's proofreading services
Discover proofreading & editing
Step 1. Ask a question
Writing a hypothesis begins with a research question that you want to answer. The question should be focused, specific, and researchable within the constraints of your project.
Step 2. Do some preliminary research
Your initial answer to the question should be based on what is already known about the topic. Look for theories and previous studies to help you form educated assumptions about what your research will find.
At this stage, you might construct a conceptual framework to ensure that you’re embarking on a relevant topic . This can also help you identify which variables you will study and what you think the relationships are between them. Sometimes, you’ll have to operationalize more complex constructs.
Step 3. Formulate your hypothesis
Now you should have some idea of what you expect to find. Write your initial answer to the question in a clear, concise sentence.
4. Refine your hypothesis
You need to make sure your hypothesis is specific and testable. There are various ways of phrasing a hypothesis, but all the terms you use should have clear definitions, and the hypothesis should contain:
- The relevant variables
- The specific group being studied
- The predicted outcome of the experiment or analysis
5. Phrase your hypothesis in three ways
To identify the variables, you can write a simple prediction in if…then form. The first part of the sentence states the independent variable and the second part states the dependent variable.
In academic research, hypotheses are more commonly phrased in terms of correlations or effects, where you directly state the predicted relationship between variables.
If you are comparing two groups, the hypothesis can state what difference you expect to find between them.
6. Write a null hypothesis
If your research involves statistical hypothesis testing , you will also have to write a null hypothesis . The null hypothesis is the default position that there is no association between the variables. The null hypothesis is written as H 0 , while the alternative hypothesis is H 1 or H a .
- H 0 : The number of lectures attended by first-year students has no effect on their final exam scores.
- H 1 : The number of lectures attended by first-year students has a positive effect on their final exam scores.
Research question | Hypothesis | Null hypothesis |
---|---|---|
What are the health benefits of eating an apple a day? | Increasing apple consumption in over-60s will result in decreasing frequency of doctor’s visits. | Increasing apple consumption in over-60s will have no effect on frequency of doctor’s visits. |
Which airlines have the most delays? | Low-cost airlines are more likely to have delays than premium airlines. | Low-cost and premium airlines are equally likely to have delays. |
Can flexible work arrangements improve job satisfaction? | Employees who have flexible working hours will report greater job satisfaction than employees who work fixed hours. | There is no relationship between working hour flexibility and job satisfaction. |
How effective is high school sex education at reducing teen pregnancies? | Teenagers who received sex education lessons throughout high school will have lower rates of unplanned pregnancy teenagers who did not receive any sex education. | High school sex education has no effect on teen pregnancy rates. |
What effect does daily use of social media have on the attention span of under-16s? | There is a negative between time spent on social media and attention span in under-16s. | There is no relationship between social media use and attention span in under-16s. |
If you want to know more about the research process , methodology , research bias , or statistics , make sure to check out some of our other articles with explanations and examples.
- Sampling methods
- Simple random sampling
- Stratified sampling
- Cluster sampling
- Likert scales
- Reproducibility
Statistics
- Null hypothesis
- Statistical power
- Probability distribution
- Effect size
- Poisson distribution
Research bias
- Optimism bias
- Cognitive bias
- Implicit bias
- Hawthorne effect
- Anchoring bias
- Explicit bias
Receive feedback on language, structure, and formatting
Professional editors proofread and edit your paper by focusing on:
- Academic style
- Vague sentences
- Style consistency
See an example
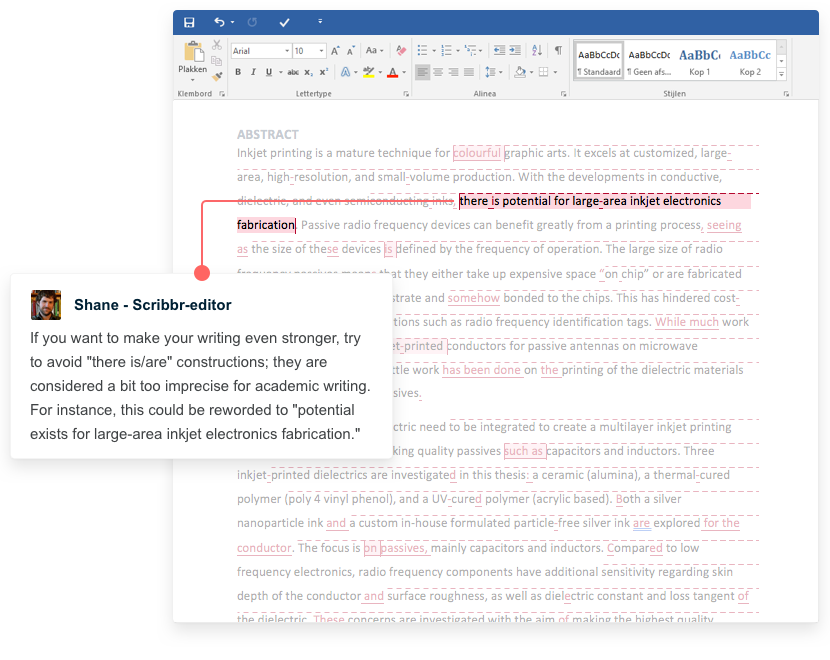
A hypothesis is not just a guess — it should be based on existing theories and knowledge. It also has to be testable, which means you can support or refute it through scientific research methods (such as experiments, observations and statistical analysis of data).
Null and alternative hypotheses are used in statistical hypothesis testing . The null hypothesis of a test always predicts no effect or no relationship between variables, while the alternative hypothesis states your research prediction of an effect or relationship.
Hypothesis testing is a formal procedure for investigating our ideas about the world using statistics. It is used by scientists to test specific predictions, called hypotheses , by calculating how likely it is that a pattern or relationship between variables could have arisen by chance.
Cite this Scribbr article
If you want to cite this source, you can copy and paste the citation or click the “Cite this Scribbr article” button to automatically add the citation to our free Citation Generator.
McCombes, S. (2023, November 20). How to Write a Strong Hypothesis | Steps & Examples. Scribbr. Retrieved September 9, 2024, from https://www.scribbr.com/methodology/hypothesis/
Is this article helpful?
Shona McCombes
Other students also liked, construct validity | definition, types, & examples, what is a conceptual framework | tips & examples, operationalization | a guide with examples, pros & cons, what is your plagiarism score.
Educational resources and simple solutions for your research journey
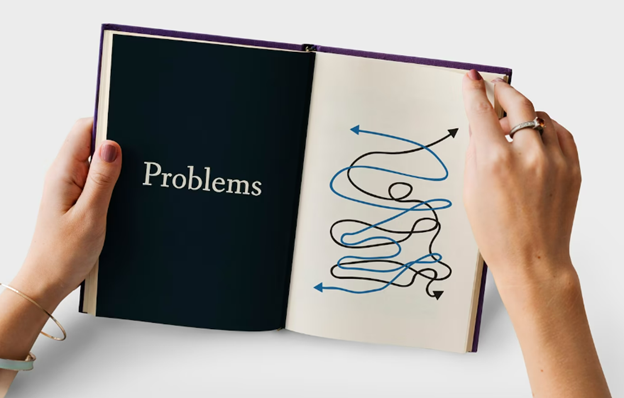
What is a Research Hypothesis: How to Write it, Types, and Examples
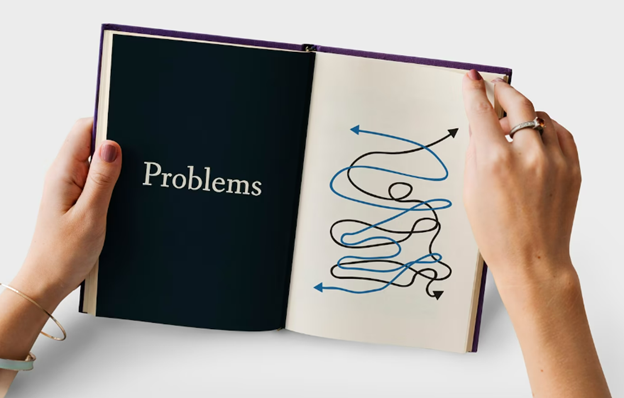
Any research begins with a research question and a research hypothesis . A research question alone may not suffice to design the experiment(s) needed to answer it. A hypothesis is central to the scientific method. But what is a hypothesis ? A hypothesis is a testable statement that proposes a possible explanation to a phenomenon, and it may include a prediction. Next, you may ask what is a research hypothesis ? Simply put, a research hypothesis is a prediction or educated guess about the relationship between the variables that you want to investigate.
It is important to be thorough when developing your research hypothesis. Shortcomings in the framing of a hypothesis can affect the study design and the results. A better understanding of the research hypothesis definition and characteristics of a good hypothesis will make it easier for you to develop your own hypothesis for your research. Let’s dive in to know more about the types of research hypothesis , how to write a research hypothesis , and some research hypothesis examples .
Table of Contents
What is a hypothesis ?
A hypothesis is based on the existing body of knowledge in a study area. Framed before the data are collected, a hypothesis states the tentative relationship between independent and dependent variables, along with a prediction of the outcome.
What is a research hypothesis ?
Young researchers starting out their journey are usually brimming with questions like “ What is a hypothesis ?” “ What is a research hypothesis ?” “How can I write a good research hypothesis ?”
A research hypothesis is a statement that proposes a possible explanation for an observable phenomenon or pattern. It guides the direction of a study and predicts the outcome of the investigation. A research hypothesis is testable, i.e., it can be supported or disproven through experimentation or observation.

Characteristics of a good hypothesis
Here are the characteristics of a good hypothesis :
- Clearly formulated and free of language errors and ambiguity
- Concise and not unnecessarily verbose
- Has clearly defined variables
- Testable and stated in a way that allows for it to be disproven
- Can be tested using a research design that is feasible, ethical, and practical
- Specific and relevant to the research problem
- Rooted in a thorough literature search
- Can generate new knowledge or understanding.
How to create an effective research hypothesis
A study begins with the formulation of a research question. A researcher then performs background research. This background information forms the basis for building a good research hypothesis . The researcher then performs experiments, collects, and analyzes the data, interprets the findings, and ultimately, determines if the findings support or negate the original hypothesis.
Let’s look at each step for creating an effective, testable, and good research hypothesis :
- Identify a research problem or question: Start by identifying a specific research problem.
- Review the literature: Conduct an in-depth review of the existing literature related to the research problem to grasp the current knowledge and gaps in the field.
- Formulate a clear and testable hypothesis : Based on the research question, use existing knowledge to form a clear and testable hypothesis . The hypothesis should state a predicted relationship between two or more variables that can be measured and manipulated. Improve the original draft till it is clear and meaningful.
- State the null hypothesis: The null hypothesis is a statement that there is no relationship between the variables you are studying.
- Define the population and sample: Clearly define the population you are studying and the sample you will be using for your research.
- Select appropriate methods for testing the hypothesis: Select appropriate research methods, such as experiments, surveys, or observational studies, which will allow you to test your research hypothesis .
Remember that creating a research hypothesis is an iterative process, i.e., you might have to revise it based on the data you collect. You may need to test and reject several hypotheses before answering the research problem.
How to write a research hypothesis
When you start writing a research hypothesis , you use an “if–then” statement format, which states the predicted relationship between two or more variables. Clearly identify the independent variables (the variables being changed) and the dependent variables (the variables being measured), as well as the population you are studying. Review and revise your hypothesis as needed.
An example of a research hypothesis in this format is as follows:
“ If [athletes] follow [cold water showers daily], then their [endurance] increases.”
Population: athletes
Independent variable: daily cold water showers
Dependent variable: endurance
You may have understood the characteristics of a good hypothesis . But note that a research hypothesis is not always confirmed; a researcher should be prepared to accept or reject the hypothesis based on the study findings.
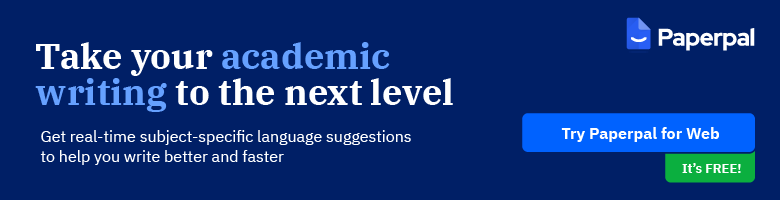
Research hypothesis checklist
Following from above, here is a 10-point checklist for a good research hypothesis :
- Testable: A research hypothesis should be able to be tested via experimentation or observation.
- Specific: A research hypothesis should clearly state the relationship between the variables being studied.
- Based on prior research: A research hypothesis should be based on existing knowledge and previous research in the field.
- Falsifiable: A research hypothesis should be able to be disproven through testing.
- Clear and concise: A research hypothesis should be stated in a clear and concise manner.
- Logical: A research hypothesis should be logical and consistent with current understanding of the subject.
- Relevant: A research hypothesis should be relevant to the research question and objectives.
- Feasible: A research hypothesis should be feasible to test within the scope of the study.
- Reflects the population: A research hypothesis should consider the population or sample being studied.
- Uncomplicated: A good research hypothesis is written in a way that is easy for the target audience to understand.
By following this research hypothesis checklist , you will be able to create a research hypothesis that is strong, well-constructed, and more likely to yield meaningful results.

Types of research hypothesis
Different types of research hypothesis are used in scientific research:
1. Null hypothesis:
A null hypothesis states that there is no change in the dependent variable due to changes to the independent variable. This means that the results are due to chance and are not significant. A null hypothesis is denoted as H0 and is stated as the opposite of what the alternative hypothesis states.
Example: “ The newly identified virus is not zoonotic .”
2. Alternative hypothesis:
This states that there is a significant difference or relationship between the variables being studied. It is denoted as H1 or Ha and is usually accepted or rejected in favor of the null hypothesis.
Example: “ The newly identified virus is zoonotic .”
3. Directional hypothesis :
This specifies the direction of the relationship or difference between variables; therefore, it tends to use terms like increase, decrease, positive, negative, more, or less.
Example: “ The inclusion of intervention X decreases infant mortality compared to the original treatment .”
4. Non-directional hypothesis:
While it does not predict the exact direction or nature of the relationship between the two variables, a non-directional hypothesis states the existence of a relationship or difference between variables but not the direction, nature, or magnitude of the relationship. A non-directional hypothesis may be used when there is no underlying theory or when findings contradict previous research.
Example, “ Cats and dogs differ in the amount of affection they express .”
5. Simple hypothesis :
A simple hypothesis only predicts the relationship between one independent and another independent variable.
Example: “ Applying sunscreen every day slows skin aging .”
6 . Complex hypothesis :
A complex hypothesis states the relationship or difference between two or more independent and dependent variables.
Example: “ Applying sunscreen every day slows skin aging, reduces sun burn, and reduces the chances of skin cancer .” (Here, the three dependent variables are slowing skin aging, reducing sun burn, and reducing the chances of skin cancer.)
7. Associative hypothesis:
An associative hypothesis states that a change in one variable results in the change of the other variable. The associative hypothesis defines interdependency between variables.
Example: “ There is a positive association between physical activity levels and overall health .”
8 . Causal hypothesis:
A causal hypothesis proposes a cause-and-effect interaction between variables.
Example: “ Long-term alcohol use causes liver damage .”
Note that some of the types of research hypothesis mentioned above might overlap. The types of hypothesis chosen will depend on the research question and the objective of the study.

Research hypothesis examples
Here are some good research hypothesis examples :
“The use of a specific type of therapy will lead to a reduction in symptoms of depression in individuals with a history of major depressive disorder.”
“Providing educational interventions on healthy eating habits will result in weight loss in overweight individuals.”
“Plants that are exposed to certain types of music will grow taller than those that are not exposed to music.”
“The use of the plant growth regulator X will lead to an increase in the number of flowers produced by plants.”
Characteristics that make a research hypothesis weak are unclear variables, unoriginality, being too general or too vague, and being untestable. A weak hypothesis leads to weak research and improper methods.
Some bad research hypothesis examples (and the reasons why they are “bad”) are as follows:
“This study will show that treatment X is better than any other treatment . ” (This statement is not testable, too broad, and does not consider other treatments that may be effective.)
“This study will prove that this type of therapy is effective for all mental disorders . ” (This statement is too broad and not testable as mental disorders are complex and different disorders may respond differently to different types of therapy.)
“Plants can communicate with each other through telepathy . ” (This statement is not testable and lacks a scientific basis.)
Importance of testable hypothesis
If a research hypothesis is not testable, the results will not prove or disprove anything meaningful. The conclusions will be vague at best. A testable hypothesis helps a researcher focus on the study outcome and understand the implication of the question and the different variables involved. A testable hypothesis helps a researcher make precise predictions based on prior research.
To be considered testable, there must be a way to prove that the hypothesis is true or false; further, the results of the hypothesis must be reproducible.
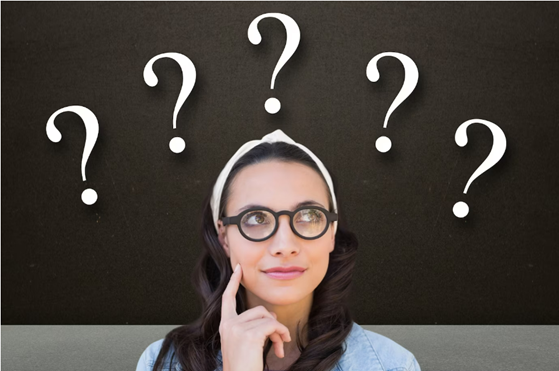
Frequently Asked Questions (FAQs) on research hypothesis
1. What is the difference between research question and research hypothesis ?
A research question defines the problem and helps outline the study objective(s). It is an open-ended statement that is exploratory or probing in nature. Therefore, it does not make predictions or assumptions. It helps a researcher identify what information to collect. A research hypothesis , however, is a specific, testable prediction about the relationship between variables. Accordingly, it guides the study design and data analysis approach.
2. When to reject null hypothesis ?
A null hypothesis should be rejected when the evidence from a statistical test shows that it is unlikely to be true. This happens when the test statistic (e.g., p -value) is less than the defined significance level (e.g., 0.05). Rejecting the null hypothesis does not necessarily mean that the alternative hypothesis is true; it simply means that the evidence found is not compatible with the null hypothesis.
3. How can I be sure my hypothesis is testable?
A testable hypothesis should be specific and measurable, and it should state a clear relationship between variables that can be tested with data. To ensure that your hypothesis is testable, consider the following:
- Clearly define the key variables in your hypothesis. You should be able to measure and manipulate these variables in a way that allows you to test the hypothesis.
- The hypothesis should predict a specific outcome or relationship between variables that can be measured or quantified.
- You should be able to collect the necessary data within the constraints of your study.
- It should be possible for other researchers to replicate your study, using the same methods and variables.
- Your hypothesis should be testable by using appropriate statistical analysis techniques, so you can draw conclusions, and make inferences about the population from the sample data.
- The hypothesis should be able to be disproven or rejected through the collection of data.
4. How do I revise my research hypothesis if my data does not support it?
If your data does not support your research hypothesis , you will need to revise it or develop a new one. You should examine your data carefully and identify any patterns or anomalies, re-examine your research question, and/or revisit your theory to look for any alternative explanations for your results. Based on your review of the data, literature, and theories, modify your research hypothesis to better align it with the results you obtained. Use your revised hypothesis to guide your research design and data collection. It is important to remain objective throughout the process.
5. I am performing exploratory research. Do I need to formulate a research hypothesis?
As opposed to “confirmatory” research, where a researcher has some idea about the relationship between the variables under investigation, exploratory research (or hypothesis-generating research) looks into a completely new topic about which limited information is available. Therefore, the researcher will not have any prior hypotheses. In such cases, a researcher will need to develop a post-hoc hypothesis. A post-hoc research hypothesis is generated after these results are known.
6. How is a research hypothesis different from a research question?
A research question is an inquiry about a specific topic or phenomenon, typically expressed as a question. It seeks to explore and understand a particular aspect of the research subject. In contrast, a research hypothesis is a specific statement or prediction that suggests an expected relationship between variables. It is formulated based on existing knowledge or theories and guides the research design and data analysis.
7. Can a research hypothesis change during the research process?
Yes, research hypotheses can change during the research process. As researchers collect and analyze data, new insights and information may emerge that require modification or refinement of the initial hypotheses. This can be due to unexpected findings, limitations in the original hypotheses, or the need to explore additional dimensions of the research topic. Flexibility is crucial in research, allowing for adaptation and adjustment of hypotheses to align with the evolving understanding of the subject matter.
8. How many hypotheses should be included in a research study?
The number of research hypotheses in a research study varies depending on the nature and scope of the research. It is not necessary to have multiple hypotheses in every study. Some studies may have only one primary hypothesis, while others may have several related hypotheses. The number of hypotheses should be determined based on the research objectives, research questions, and the complexity of the research topic. It is important to ensure that the hypotheses are focused, testable, and directly related to the research aims.
9. Can research hypotheses be used in qualitative research?
Yes, research hypotheses can be used in qualitative research, although they are more commonly associated with quantitative research. In qualitative research, hypotheses may be formulated as tentative or exploratory statements that guide the investigation. Instead of testing hypotheses through statistical analysis, qualitative researchers may use the hypotheses to guide data collection and analysis, seeking to uncover patterns, themes, or relationships within the qualitative data. The emphasis in qualitative research is often on generating insights and understanding rather than confirming or rejecting specific research hypotheses through statistical testing.
Editage All Access is a subscription-based platform that unifies the best AI tools and services designed to speed up, simplify, and streamline every step of a researcher’s journey. The Editage All Access Pack is a one-of-a-kind subscription that unlocks full access to an AI writing assistant, literature recommender, journal finder, scientific illustration tool, and exclusive discounts on professional publication services from Editage.
Based on 22+ years of experience in academia, Editage All Access empowers researchers to put their best research forward and move closer to success. Explore our top AI Tools pack, AI Tools + Publication Services pack, or Build Your Own Plan. Find everything a researcher needs to succeed, all in one place – Get All Access now starting at just $14 a month !
Related Posts

How to Write a Dissertation: A Beginner’s Guide

Back to School – Lock-in All Access Pack for a Year at the Best Price

An official website of the United States government
The .gov means it’s official. Federal government websites often end in .gov or .mil. Before sharing sensitive information, make sure you’re on a federal government site.
The site is secure. The https:// ensures that you are connecting to the official website and that any information you provide is encrypted and transmitted securely.
- Publications
- Account settings
Preview improvements coming to the PMC website in October 2024. Learn More or Try it out now .
- Advanced Search
- Journal List

Great Ape Genomics
The great ape families are the species most closely related to our own, comprising chimpanzees, bonobos, gorillas, and orangutans. They live exclusively in tropical rainforests in Central Africa and the islands of Southeast Asia. Due to their close evolutionary relationship with humans, great apes share many cognitive, physiological, and morphological similarities with humans. The members of the great ape family make obvious models to facilitate the further understanding about humans' biology and history. This review will discuss how the recent addition of genome-wide data from great apes has furthered humans' understanding of these species and humanity, especially in the realm of evolutionary genetics.
Introduction
It has long been hypothesized that humans and the great apes share a recent common ancestry ( Huxley 1863 ; Darwin 1871 ). This result has been confirmed by many molecular studies over the past several decades ( Sibley and Ahlquist 1987 ; Goodman 1996 ; Satta et al. 2000 ; Chen and Li 2001 ). It is now known that common chimpanzees ( Pan troglodytes ) and bonobos ( Pan paniscus ) are our closest living relatives, followed by gorillas and orangutans (Figure 1 ). The sequence divergence at orthologous sites between humans and chimpanzees/bonobos is just 1.2% ( Chimpanzee Sequencing and Analysis Consortium 2005 ; Prufer et al. 2012 ), which is much less than the divergence between two random fruit flies ( Drosophila simulans ) of the same species ( Begun et al. 2007 ). Great apes are studied by geneticists for a wide variety of reasons, including intrinsic biological interest, interest in the horizontal transfer of pathogens such as HIV-1 ( Keele et al. 2006 ), implications for conservation biology, and interest in recently evolved human-specific traits such as bipedality and large, complex brains. With assembled genome sequences now available from four different great ape species ( Chimpanzee Sequencing and Analysis Consortium 2005 ; Locke et al. 2011 ; Prufer et al. 2012 ; Scally et al. 2012 ), as well as 100 additional genomes available for population genomic studies ( Locke et al. 2011 ; Auton et al. 2012 ; Prado-Martinez et al. 2013 ), there is now an unprecedented ability to study the evolution of humanity's closest relatives. This review highlights how recent comparative studies have enhanced the knowledge of human genetics and human evolution. A comprehensive review is beyond the scope of this paper; rather, it highlights specific topics that represent major areas of current research.

Schematic showing the evolutionary relationships and approximate divergence times among the great apes and humans. Ppy, Pongo pygmaeus ; Pab, P. abelii ; Ggo, Gorilla gorilla ; Gbe, G. beringei ; Hsa, Homo sapiens ; Ppa, Pan paniscus ; Ptr, P. troglodytes ; Mya, Million years ago.
How Are Humans Unique?
By aligning the human reference sequence to the genomes of several closely related outgroup species, we can identify mutations that have occurred on the recent human branch and distinguish between ancestral and derived alleles at human single nucleotide polymorphisms (SNPs). Over the whole genome, these mutations occur at a roughly constant rate, consistent with the idea that the vast majority of substitutions are selectively neutral (i.e., nonfunctional) ( Kimura 1983 ). Orangutans with a 2.4-fold higher sequence divergence (compared with chimpanzees) are expected to have a 2.4-fold longer divergence time from humans, in relation to the human-chimpanzee divergence time (Figure 1 ). Part of the challenge of identifying the genetic basis of human-specific morphological change is the difficulty in distinguishing between rare functional mutations and the more common selectively neutral mutations that separate humans from the great apes. For obvious ethical reasons, function can generally not be inferred in vivo. Rather, indirect computational techniques, as well as the utilization of a more distantly related animal model (e.g., mice and fruit flies) have been used to identify human-specific adaptations.
Coding Changes
One approach for identifying human-specific adaptions is to scan the exomes of humans (and various outgroups) to identify genes with patterns of genetic variation that are consistent with the action of recent natural selection. For example, Enard and colleagues ( Enard et al. 2002 ) sequenced the FOXP2 gene, a forkhead class transcription factor, in humans, great apes, and mice. The gene is highly conserved among mammals, with just one non-synonymous (i.e., amino-acid changing) mutation separating the chimpanzee and mouse coding sequences. This conservation is not due to a low mutation rate, as there are 147 synonymous (i.e., non-amino acid changing) mutations between chimpanzees and mice. However, on the human-specific branch, there are two non-synonymous mutations and no synonymous mutations, which is significantly different from expectations (p<10 −3 , Fisher's exact test). Enard and colleagues interpreted this (and other evidence) as support for recent positive selection in the human lineage ( Enard et al. 2002 ). Since mutations in FOXP2 can cause deficiencies in spoken language capacity, Enard and cohort speculated that the non-synonymous mutations in FOXP2 were related to humans' ability to develop complex forms of communication. Subsequent work has shown that both Neanderthals and Denisovans also contain these two recent non-synonymous mutations ( Krause et al. 2007 ; Reich et al. 2010 ), though this observation can be interpreted in different ways ( Coop et al. 2008 ; Ptak et al. 2009 ). Recent work has suggested that a mutation at a transcription factor binding site in intron 8 of the FOXP2 gene affects FOXP2 expression levels. These expression levels might have been recently selected for because they differ between modern humans and Neanderthals ( Maricic et al. 2012 ).
Subsequently, researchers have searched more systematically through many different genes to look for a similar signal of increased human-specific, non-synonymous substitutions relative to synonymous substitutions; first using unrooted trees ( Clark et al. 2003 ; Nielsen et al. 2005 ) and later with one or more outgroups ( Rhesus Macaque Genome Sequencing and Analysis Consortium 2007 ; Lindblad-Toh et al. 2011 ). These studies have identified functional categories (i.e., the genes involved in spermatogenesis) that are enriched in genes showing evidence for positive selection, but the biological insight gained from these broad surveys is somewhat limited. Deeper insight generally requires additional information from physiological, behavioral, or targeted genetic studies. For example, great ape species differ in the size and structure of their social groups—chimpanzees live in multi-male, multi-female groups; gorillas live in single male, multi-female groups; and orangutans are mostly solitary. These life-history traits suggest that different great ape species undergo different sexual selection pressures. In particular, since receptive chimpanzee females mate with multiple males (unlike gorilla and orangutan females), it is expected that sperm competition will be more important for chimpanzee males than for males of other great ape species. Both physiological ( Harcourt et al. 1981 ) and genetic ( Dorus et al. 2004 ) studies provide support for this hypothesis, and may provide a partial explanation for the prevalence of genes that are involved in spermatogenesis that appear to be affected by recent positive selection.
Another approach for detecting the action of recent natural selection on the human lineage involves comparing synonymous (i.e., non-amino-acid changing) versus non-synonymous (i.e., amino-acid changing) polymorphisms and substitutions. If a gene has been subject to recent positive (i.e., adaptive) selection, this can lead to the fixation of several non-synonymous mutations, and an excess of non-synonymous substitutions relative to non-synonymous polymorphisms ( McDonald and Kreitman 1991 ). Conversely, under continued purifying selection most non-synonymous mutations will be deleterious, and very few will rise in frequency to become fixed differences. This will tend to produce a deficit of non-synonymous substitutions relative to non-synonymous polymorphisms. Bustamante and colleagues examined the patterns of polymorphism and divergence in thirty-nine humans (20 European-Americans and 19 African-Americans) and one chimpanzee at more than 11,000 different protein-coding genes ( Bustamante et al. 2005 ). As with other studies, they found certain functional categories were overrepresented (or underrepresented) among genes that they had inferred to be recently selected for, but functional insight for specific genes was minimal.
Regulatory Changes
One potential limitation of the studies described above is that they are confined to protein-coding regions, which comprise <2% of the human genome. It is possible that most of the genetic changes that “make us human” involve regulatory sequences rather than coding sequences ( King and Wilson 1975 ). To identify the noncoding regions that are important to recent human evolution, Pollard, Siepel, and colleagues developed a likelihood-ratio test to estimate the statistical significance of regions that show an apparent increase in the substitution rate on the human evolutionary branch ( Siepel, et al. 2006 ). Siepel and cohort then applied this method to whole genome sequence data from humans, and several other vertebrates, to identify 202 regions that showed significant rates of human-specific evolution ( Pollard, Salama, King et al. 2006 ), which they called human accelerated regions (HARs). The most significant of these regions, HAR1, has 18 substitutions in 118 base pairs (relative to chimpanzees) and contains part of a novel RNA gene that is expressed in the human neocortex from seven to nineteen gestational weeks ( Pollard, Salama, Lambert et al. 2006 ). Though the specific function of HAR1 is not known, it is tempting to speculate that it is related to the extreme growth in size and complexity of human brains in relation to other apes.
Pollard, Salama, King et al. (2006) found the substitutions at HARs show a weak-to-strong bias (i.e., tend to be from A or T to G or C), and that HARs tend to be in regions of high recombination. Both of these observations are consistent with the possibility that GC-biased gene conversion may play an important role in the creation of HARs ( Galtier and Duret 2007 ; Katzman et al. 2010 ).
A complementary approach to identifying important regulatory changes involves analyses of gene expression levels using microarrays, or large-scale sequencing of RNA (RNA-seq) using next-generation sequencing ( Romero et al. 2012 ). One intriguing hypothesis is that humans' unique cognitive abilities are due to changes in gene expression patterns in the brain during development ( Caceres et al. 2003 ; Nowick et al. 2009 ; Xu et al. 2010 ), and there is some evidence in favor of this idea ( Somel et al. 2009 ). More generally, measurements of gene expression within individuals of the same species, and between individuals of different species, are informative about the current or past action of natural selection for specific expression levels. For example, if between-species levels of gene expression variation are no larger than within-species levels, stabilizing selection on gene regulation is a likely explanation ( Gilad et al. 2006 ). In contrast, if a higher expression level is observed specifically in one species, then directional selection for differential expression in that species is most likely operating. Recent studies have shown that the regulation of a majority of the genes in the genome evolve under evolutionary constraint, consistent with widespread stabilizing selection ( Lemos et al. 2005 ; Rifkin et al. 2005 ). In addition, a substantial fraction of human and great ape genes show species-specific changes in gene expression levels that are best explained by recent positive selection ( Gilad et al. 2006 ; Blekhman et al. 2008 ). Finally, it is important to note that gene expression levels are only an intermediate phenotype, and evidence of differential expression across species provides only a starting point for targeted studies to determine the genetic mechanism causing the difference and the function consequences (if any).
Gain or Loss of Genes
A related approach focuses on identifying and characterizing the gain or loss of genes in the human lineage subsequent to the divergence of humans and chimpanzees. Olson proposed that gene loss could be an important mechanism in the evolution of human-specific traits ( Olson 1999 ). However, further studies have found that the rate of gene loss in the human-specific lineage is the same as the comparable rate in other great ape lineages ( Kim et al. 2010 ; Prado-Martinez et al. 2013 ), suggesting that humans are not unusual in their rate of genic turnover. While this does not disprove Olson's hypothesis, it suggests that whatever role gene loss plays in species-specific traits is likely to be a general (rather than human-specific) phenomenon. In a few cases, more detailed studies have highlighted potential selective explanations for complete ( Stedman et al. 2004 ) or partial ( Xue et al. 2006 ) loss of genes in humans. Conversely, while several human-specific genes have been identified ( Knowles and McLysaght 2009 ; Wu et al. 2011 ), most of these genes are of unknown function (but see [ Buhl et al. 2006 ; Hu et al. 2012 ]), making their relevance to recent human evolution difficult to quantify.
Structural Changes
Humans have a diploid chromosome number of 2n = 46, while the great apes have 2n = 48 chromosomes ( Yunis and Prakash 1982 ). Evolutionary genetic studies of the human chromosome 2 have highlighted additional evidence that this chromosome was formed by a fusion of two autosomes within the past several million years ( Dreszer et al. 2007 ; Auton et al. 2012 ; Ventura et al. 2012 ). Additional human-specific inversions ( Yunis and Prakash 1982 ) and copy number variations ( Dumas et al. 2007 ) were identified by conventional banding techniques, while more recent array-based comparative genomic hybridization experiments ( Linardopoulou et al. 2005 ; Goidts et al. 2006 ; Wilson et al. 2006 ) and next-generation sequencing studies ( Alkan et al. 2009 ) have identified many more large rearrangements and segmental duplications that are unique to the human lineage. When all forms of variability are taken into account, the estimated divergence between humans and chimpanzees increases from 1.2% (single nucleotide polymorphisms at aligned orthologous sites) to ∼5% ( Britten 2002 ). It is unclear what, if any, functional relevance many of these structural changes have in humans, though it may be relevant that regions near cytogenetically visible changes in the human genome are enriched for recent gene duplications and new genes ( Fortna et al. 2004 ; Dumas et al. 2007 ).
Comparative Demography
The amount of divergence between two DNA sequences is, on average, directly proportional to the time since they last shared a common ancestor. By comparing patterns of genetic variation across multiple individuals from different populations, it is possible to make inferences about a species' demographic history including population migration, changes in population size, geographic structure, and admixture between diverged populations. By inferring demographic parameters in both humans and great apes, it can be determined if particular observations that are found in one species are common or unusual. This, in turn, allows for a more realistic assessment of the ways in which the human species might be unique.
Population Size
The current census size for the human population tops out at more than 7 billion individuals and continues to grow very rapidly. However, it is known that even as recently as several centuries ago, the human population size was orders of magnitude smaller. In fact, genetic studies have consistently found that two randomly chosen human chromosomes tend to differ from each other at roughly 1 in a 1000 base pairs, which is consistent with a long-term effective population size (N e ) of 10,000–15,000 ( Li and Sadler 1991 ; Harding et al. 1997 ; Frisse et al. 2001 ). Here, N e refers to the size of a randomly mating population that is expected to have the same levels of genetic diversity as what is observed. Although there are many reasons why N e might be less than the census population size ( Caballero 1994 ), the difference in magnitude is still immense, and requires explanation. The current belief is that for much of human history, the population sizes were much smaller, and it is only very recently (i.e., within the past 20-30 thousand years) that the population sizes have increased rapidly ( Pluzhnikov et al.2002 ; Voight et al. 2005 ; Gutenkunst et al. 2009 ). Recent studies have identified an excess of very rare single nucleotide variants (minor allele frequency<0.1 %), consistent with explosive population growth over the past several thousand years, starting subsequent to the development and spread of agriculture ( Coventry et al. 2010 ; Nelson et al. 2012 ).
In contrast, extant great apes are found exclusively in Old World tropical rain forests, and their ranges (and estimated census population sizes) have decreased dramatically over the past 200 years ( Junker et al. 2012 ). While initial estimates of great ape genetic diversity from microsatellites were low ( Wise et al. 1997 ; Cooper et al. 1998 ), subsequent studies of nuclear sequence variation have found that all great ape species, except for bonobos, have levels of genetic variation substantially higher than the levels of human genetic variation ( Kaessmann et al. 2001 ; Fischer et al. 2006 ; Locke et al. 2011 ; Prado-Martinez et al. 2013 ). Since the underlying mutation rates are unlikely to be different between humans and great apes, the differences can be attributed to chimpanzees, gorillas, and orangutans having larger long-term N e 's than humans have. This finding highlights the value of a comparative demographic approach, as it emphasizes that the current human dominance (both in range and population size) is very recent—even though humans' ancestors had populated Eurasia and Africa for almost 2 million years, while the great apes have been confined to a much smaller area during that time. Most likely, there were more chimpanzees, gorillas, and orangutans around at any time prior to 50,000 years ago than there were humans.
Studies that estimate ancestral (effective) population sizes (N a ) provide additional evidence that humans' current N e is unusually low. Takahata (1993) examined genetic diversity in the human major histocompatibility complex (MHC), and concluded that the diversity was consistent with a N a of ∼ 100,000. Other approaches, such as using the variance in human-chimpanzee divergence across the genome, the discordance of gene trees across the genome, or maximum-likelihood techniques, have estimated N a ≥50,000 for the population ancestral to humans and chimpanzees ( Takahata and Satta 1997 ; Chen and Li 2001 ; O'hUigin et al. 2002 ; Wall 2003 ; Burgess and Yang 2008 ). While estimates of N a can be inflated due to past population structure, the basic picture appears to be that almost all extant and ancestral ape populations have (or had) substantially more genetic variation (and larger effective population sizes) than extant modern humans.
Population Structure
An additional way of viewing the low N e in humans is the idea that all extant people are genetically similar when compared with the great apes. Another facet of this idea is that all great apes consist of groups of diverged populations that are taxonomically considered to be separate species or sub-species ( Groves 2006 ). Currently, there are the chimpanzee and bonobo ( P. troglodytes and P. paniscus respectively), two gorilla species ( Gorilla gorilla and Gorilla beringei ), and two orangutan species ( Pongo pygmaeus and Pongo abelii ), and additional subspecies from each group, compared with just a single extant species and subspecies of Homo sapiens sapiens . Sister great ape species within the same genus can interbreed and produce fertile offspring in zoos, but not in the wild where their ranges generally do not overlap. The taxonomic differences between great ape populations are not purely semantic though, in that the genetic distance between great ape species is substantially larger than the genetic distance between isolated human groups ( Fischer et al. 2006 ; Wall et al. 2008 ). Broadly speaking, the existence of multiple diverged populations that can admix and interbreed is the general pattern in catarrhine primates, including gibbons, macaques, baboons, vervets, and guenons ( Jolly 2001 ; Arnold and Meyer 2006 ), with humans and a few isolated species (e.g., the siamang) as outliers. It is unclear why this is so, but perhaps some aspect of life-history behavior, mate choice, or migration facilitates the isolation (and genetic divergence) of nearby populations. Interestingly, just 50,000 years ago, the human species coexisted with at least three other archaic human groups, including Neanderthals, Denisovans, and Homo floresiensis ( Wall and Slatkin 2012 ), and potentially several other diverged populations ( Harvati et al. 2011 ; Curnoe et al. 2012 ). H. floresiensis coexisted with modern humans in island Southeast Asia as recently as 12,000 years ago ( Morwood et al. 2005 ). Perhaps not coincidentally, these archaic human groups all disappeared around the same time as modern human populations started to increase rapidly in size.
X Versus Autosome Comparisons
Comparative studies of humans and great apes can also shed light on sex-specific differences, and life-history traits in each species. In a randomly mating population with equal numbers of males and females, there are three X chromosomes for every four autosomes, leading to a 3:4 ratio in expected levels of diversity in X versus autosome comparisons. If a species is generally polygynous, there will be more breeding females than breeding males, which tends to increase the ratio of X-to-autosome diversity ( Caballero 1994 ). Gorillas, who are strongly polygynous, are expected to have increased relative levels of X-linked diversity when compared with humans (weakly polygynous) and gibbons (monogamous). Concurrently recent positive selection, which decreases levels of genetic variation, is expected to affect the X chromosome more than the autosomes due to hemizygosity in males and the recessivity of some advantageous alleles. Analyses of human polymorphism data show the effects of both of these evolutionary processes ( Hammer et al. 2008 ; Hammer et al. 2010 ; Keinan et al. 2009 ). Overall, the ratio of X-to-autosome diversity is much less than 0.75, which is consistent with the stronger effect of selection on the X chromosome. However, if the analysis is confined to regions far away from genes, where natural selection plays less of a role, the situation is reversed, with X-to-autosome ratios significantly higher than 0.75. A full analysis of whole genome sequencing data from the Great Ape Genomes Project ( Prado-Martinez et al. 2013 ) is currently in process, and the results will be informative about the relative roles of natural selection, mating system, and sex-specific migration in shaping genome-wide patterns of genetic variation.
Comparative Population Genetics
As hinted at above, between-species comparisons can also be informative about other population genetic processes such as natural selection, mutation, and recombination. Two examples are discussed in greater detail below.
Recombination rates vary dramatically between individuals and between different regions of the genome. In particular, it is now well established that most recombination events occur in narrow 1–2 kilobase (kb) wide “hot spots”, with almost two-thirds of all crossovers occurring in 10% of the human genome ( Myers et al. 2005 ). Roughly 40% of these recombination hot spots contain a (partially degenerate) thirteen base pair sequence motif, which matches the predicted binding site of the PRDM9 gene, and is thought to be involved in double strand break formation ( Baudat et al. 2010 ; Myers et al. 2010 ). PRDM9 evolves rapidly in metazoans ( Oliver et al. 2009 ), and specific single nucleotide variants have been shown to strongly affect male recombination rates ( Berg et al. 2010 ).
Recent studies of recombination in chimpanzees have shown that humans and chimpanzees generally do not share recombination hot spots ( Wall et al. 2003 ; Ptak et al. 2004 ; Winckler et al. 2005 ; Auton et al. 2012 ), but most hot spots are shared across different human populations. Further, there is not a simple sequence motif that appears to be associated with the location of chimpanzee hot spots ( Auton et al. 2012 ). This situation is not surprising, since there is a single dominant PRDM9 allele at high frequency in humans (corresponding to the thirteen base pair [bp] sequence motif found near human recombination hot spots) but many low frequency PRDM9 alleles in chimpanzees. Even if PRDM9 plays a primary role in hot spot localization, it appears that the allelic diversity at PRDM9 in chimpanzees promotes a wide array of different hot spot locations in different chimpanzee individuals. Since large-scale detection of recombination hot spots is based on patterns of linkage disequilibrium, which reflect long-term average rates of recombination, it is unlikely that a particular sequence motif will be associated with hot spots unless there is a dominant (i.e., high-frequency) PRDM9 allele. It remains to be seen how common hot spot motifs are, but ongoing studies of recombination rates in all great ape species will provide a partial answer to this question (L Stevison and J Wall, unpublished data).
Whole genome sequence polymorphism data in great apes also help facilitate the search for polymorphisms that are shared by descent between multiple species. Theory suggests that such ancient shared polymorphisms are unlikely to arise by chance ( Leffler et al. 2013 ), and are more likely due to long-lived balancing or frequency-dependent selection (e.g., selection for rare alleles, or selection for heterozygotes). One well-known example of ancient balancing selection is from the MHC, which has a primary role in the active immune system ( Klein et al. 1993 ). Mutations in the MHC have been shown to be present before the split of humans from the great apes, and to persist as polymorphisms in multiple species due to selection favoring heterozygous genotypes for over 12 million years ( Ayala 1995 ). Beyond this one well-known example, the evidence for ancient balancing selection is rare, in part because of the difficulty in distinguishing mutations that are identical by descent (i.e., arose before the split of humans and chimpanzees) from those that are identical by state (i.e., parallel mutations that happened at the same nucleotide site in multiple species). Whole genome sequence data makes it possible to distinguish between the two by looking for multi-SNP haplotypes shared across species ( Leffler et al. 2013 ). Leffler and colleagues utilized complete genome sequences from fifty-nine humans and ten chimpanzees to identify 125 regions with haplotypes shared between humans and chimpanzees. These regions are significantly enriched for membrane glycoproteins, suggesting that genes involved in host-pathogen interactions are more likely to have been subject to ancient balancing selection. This idea is in keeping with previous studies of ancient balancing selection in humans ( Klein et al. 1993 ; Segurel et al. 2012 ) as well as more recent balancing selection in humans and other species ( Stahl et al. 1999 ; Hedrick 2011 ).
Great Apes as Reservoirs of Disease
One area where great ape genomics is of direct relevance to human health involves infectious diseases that are shared across species (i.e., zoonosis). The most well known example concerns HIV-1, a strain of human immunodeficiency virus that is pandemic in humans ( Sharp and Hahn 2011 ). Initial genetic studies of HIV found that the disease was closely related to other lentiviruses found in African apes and monkeys. It has been shown that HIV-1 is similar to SIVcpz, a simian immunodeficiency virus found in the central chimpanzee Pan troglodytes troglodytes ( Huet et al. 1990 ). Subsequent studies of natural chimpanzee populations confirmed P. t. troglodytes as a natural reservoir of HIV-1, and indicated that there had been multiple transmission events of HIV from chimpanzees to humans ( Keele et al. 2006 ). Chimpanzees in turn are thought to have acquired SIVcpz relatively recently from eating infected monkeys (mangabeys, guenons, or both). There is some evidence that chimpanzees infected with SIVcpz can develop AIDS ( Sharp and Hahn 2011 ), but there is a possibility that studies of how chimpanzees (and gorillas who are infected by SIVgor) physiologically respond to SIV may provide some insight into how humans can treat current HIV-1 infections.
Conservation Biology
One of the primary motivations for studying great ape demography is to help inform advocacy and policy decisions for promoting the conservation, health, and genetic diversity of our evolutionary cousins. All great ape species are considered to be Endangered or Critically Endangered by the International Union for Conservation of Nature ( IUCN 2012 ) due to the recent drastic reduction of their rainforest habitat ( Junker et al. 2012 ). From a conservation perspective, the priority is obviously to increase (or maintain) suitable habitats for great apes, and to eliminate (or reduce) human-mediated mortality, but there is an important role for genomics in helping conservation biologists focus their efforts. DNA-based studies can help identify populations that are genetically distinct from all others, which can elevate the importance of preserving them as a previously unknown reservoir of genetic diversity. For example, a study of orangutan mtDNA helped the recognition of the Sumatran orangutan as a separate species ( Xu and Arnason 1996 ), and a study of autosomal SNP variation argued for the creation of a fourth chimpanzee subspecies, Pan troglodytes ellioti ( Bowden et al. 2012 ). Genetic studies have also estimated the extent of recent population decline in orangutans ( Goossens et al. 2006 ) and chimpanzees ( Campbell et al. 2008 ), and identified important evidence for long-range migration in the Sumatran orangutan ( Nater et al. 2013 ). Finally, additional analyses are possible with widespread whole-genome sequence data in great apes. To some extent, the provenance of captive animals can be inferred from large-scale genetic data, and recent work suggests that most captive individuals are genetically admixed from two, or more, distinct wild-born populations ( Prado-Martinez et al. 2013 ). Prado-Martinez and colleagues also utilized runs of homozygosity ( McQuillan et al. 2008 ) to quantify the extent of inbreeding in different great ape species. Bonobos had large homozygous tracts similar to isolated human groups that are known to have small population sizes (e.g., the Karitiana from Brazil), suggesting that inbreeding is an ongoing concern in extant bonobo populations.
Conclusions
Given the dire situation that all great ape populations currently face, there is added motivation for researchers to learn as much as possible from their genomes, both to provide additional justification for conservation efforts and because such studies may be impossible in the not-too-distant future. As highlighted above, great ape genomic studies have already been highly informative in regards to human biology, human history, and the effects of natural selection. The great apes are likely to remain of critical importance for future human genetic studies, even without the benefits of manipulative studies that are used in model organisms such as the macaque or the mouse.
Acknowledgments
This work was supported in part by NIH grant R01 HG005226.
- Alkan C, Kidd JM, Marques-Bonet T, Aksay G, Antonacci F, Hormozdiari F, Kitzman JO, Baker C, Malig M, Mutlu O, Sahinalp SC, Gibbs RA, Eichler EE. Personalized copy number and segmental duplication maps using next-generation sequencing. Nat Genet. 2009; 41 :1061–1067. [ PMC free article ] [ PubMed ] [ Google Scholar ]
- Arnold ML, Meyer A. Natural hybridization in primates: One evolutionary mechanism. Zoology (Jena) 2006; 109 :261–276. [ PubMed ] [ Google Scholar ]
- Auton A, Fledel-Alon A, Pfeifer S, Venn O, Segurel L, Street T, Leffler EM, Bowden R, Aneas I, Broxholme J, Humburg P, Iqbal Z, Lunter G, Maller J, Hernandez RD, Melton C, Venkat A, Nobrega MA, Bontrop R, Myers S, Donnelly P, Przeworski M, McVean G. A fine-scale chimpanzee genetic map from population sequencing. Science. 2012; 336 :193–198. [ PMC free article ] [ PubMed ] [ Google Scholar ]
- Ayala FJ. The myth of Eve: Molecular biology and human origins. Science. 1995; 270 :1930–1936. [ PubMed ] [ Google Scholar ]
- Baudat F, Buard J, Grey C, Fledel-Alon A, Ober C, Przeworski M, Coop G, de Massy B. PRDM9 is a major determinant of meiotic recombination hotspots in humans and mice. Science. 2010; 327 :836–840. [ PMC free article ] [ PubMed ] [ Google Scholar ]
- Begun DJ, Holloway AK, Stevens K, Hillier LW, Poh YP, Hahn MW, Nista PM, Jones CD, Kern AD, Dewey CN, Pachter L, Myers E, Langley CH. Population genomics: Whole-genome analysis of polymorphism and divergence in Drosophila simulans. PLoS Biol. 2007; 5 e310. [ PMC free article ] [ PubMed ] [ Google Scholar ]
- Berg IL, Neumann R, Lam KW, Sarbajna S, Odenthal-Hesse L, May CA, Jeffreys AJ. PRDM9 variation strongly influences recombination hot-spot activity and meiotic instability in humans. Nat Genet. 2010; 42 :859–863. [ PMC free article ] [ PubMed ] [ Google Scholar ]
- Blekhman R, Oshlack A, Chabot AE, Smyth GK, Gilad Y. Gene regulation in primates evolves under tissue-specific selection pressures. PLoS Genet. 2008; 4 e1000271. [ PMC free article ] [ PubMed ] [ Google Scholar ]
- Bowden R, MacFie TS, Myers S, Hellenthal G, Nerrienet E, Bontrop RE, Freeman C, Donnelly P, Mundy NI. Genomic tools for evolution and conservation in the chimpanzee: Pan troglodytes ellioti is a genetically distinct population. PLoS Genet. 2012; 8 e1002504. [ PMC free article ] [ PubMed ] [ Google Scholar ]
- Britten RJ. Divergence between samples of chimpanzee and human DNA sequences is 5%, counting indels. Proc Natl Acad Sci U S A. 2002; 99 :13633–13635. [ PMC free article ] [ PubMed ] [ Google Scholar ]
- Buhl AM, Jurlander J, Jorgensen FS, Ottesen AM, Cowland JB, Gjerdrum LM, Hansen BV, Leffers H. Identification of a gene on chromosome 12q22 uniquely overexpressed in chronic lymphocytic leukemia. Blood. 2006; 107 :2904–2911. [ PubMed ] [ Google Scholar ]
- Burgess R, Yang Z. Estimation of hominoid ancestral population sizes under bayesian coalescent models incorporating mutation rate variation and sequencing errors. Mol Biol Evol. 2008; 25 :1979–1994. [ PubMed ] [ Google Scholar ]
- Bustamante CD, Fledel-Alon A, Williamson S, Nielsen R, Hubisz MT, Glanowski S, Tanenbaum DM, White TJ, Sninsky JJ, Hernandez RD, Civello D, Adams MD, Cargill M, Clark AG. Natural selection on protein-coding genes in the human genome. Nature. 2005; 437 :1153–1157. [ PubMed ] [ Google Scholar ]
- Caballero A. Developments in the prediction of effective population size. Heredity (Edinb) 73 (Pt 6) 1994:657–679. [ PubMed ] [ Google Scholar ]
- Caceres M, Lachuer J, Zapala MA, Redmond JC, Kudo L, Geschwind DH, Lockhart DJ, Preuss TM, Barlow C. Elevated gene expression levels distinguish human from non-human primate brains. Proc Natl Acad Sci U S A. 2003; 100 :13030–13035. [ PMC free article ] [ PubMed ] [ Google Scholar ]
- Campbell G, Kuehl H, N'Goran Kouame P, Boesch C. Alarming decline of West African chimpanzees in Cote d'Ivoire. Curr Biol. 2008; 18 :R903–904. [ PubMed ] [ Google Scholar ]
- Chen FC, Li WH. Genomic divergences between humans and other hominoids and the effective population size of the common ancestor of humans and chimpanzees. Am J Hum Genet. 2001; 68 :444–456. [ PMC free article ] [ PubMed ] [ Google Scholar ]
- Clark AG, Glanowski S, Nielsen R, Thomas PD, Kejariwal A, Todd MA, Tanenbaum DM, Civello D, Lu F, Murphy B, Ferriera S, Wang G, Zheng X, White TJ, Sninsky JJ, Adams MD, Cargill M. Inferring nonneutral evolution from human-chimp-mouse orthologous gene trios. Science. 2003; 302 :1960–1963. [ PubMed ] [ Google Scholar ]
- Chimpanzee Sequencing and Analysis Consortium. Initial sequence of the chimpanzee genome and comparison with the human genome. Nature. 2005; 437 :69–87. [ PubMed ] [ Google Scholar ]
- Coop G, Bullaughey K, Luca F, Przeworski M. The timing of selection at the human FOXP2 gene. Mol Biol Evol. 2008; 25 :1257–1259. [ PMC free article ] [ PubMed ] [ Google Scholar ]
- Cooper G, Rubinsztein DC, Amos W. Ascertainment bias cannot entirely account for human microsatellites being longer than their chimpanzee homologues. Hum Mol Genet. 1998; 7 :1425–1429. [ PubMed ] [ Google Scholar ]
- Coventry A, Bull-Otterson LM, Liu X, Clark AG, Maxwell TJ, Crosby J, Hixson JE, Rea TJ, Muzny DM, Lewis LR, Wheeler DA, Sabo A, Lusk C, Weiss KG, Akbar H, Cree A, Hawes AC, Newsham I, Varghese RT, Villasana D, Gross S, Joshi V, Santibanez J, Morgan M, Chang K, Iv WH, Templeton AR, Boerwinkle E, Gibbs R, Sing CF. Deep resequencing reveals excess rare recent variants consistent with explosive population growth. Nat Commun. 2010; 1 :131. [ PMC free article ] [ PubMed ] [ Google Scholar ]
- Curnoe D, Xueping J, Herries AI, Kanning B, Tacon PS, Zhende B, Fink D, Yunsheng Z, Hellstrom J, Yun L, Cassis G, Bing S, Wroe S, Shi H, Parr WC, Shengmin H, Rogers N. Human remains from the Pleistocene-Holocene transition of southwest China suggest a complex evolutionary history for East Asians. PLoS One. 2012; 7 e31918. [ PMC free article ] [ PubMed ] [ Google Scholar ]
- Darwin C. London, England: John Murray; 1871. The Descent of Man, and Selection in Relation to Sex. [ Google Scholar ]
- Dorus S, Evans PD, Wyckoff GJ, Choi SS, Lahn BT. Rate of molecular evolution of the seminal protein gene SEMG2 correlates with levels of female promiscuity. Nat Genet. 2004; 36 :1326–1329. [ PubMed ] [ Google Scholar ]
- Dreszer TR, Wall GD, Haussler D, Pollard KS. Biased clustered substitutions in the human genome: The footprints of male-driven biased gene conversion. Genome Res. 2007; 17 :1420–1430. [ PMC free article ] [ PubMed ] [ Google Scholar ]
- Dumas L, Kim YH, Karimpour-Fard A, Cox M, Hopkins J, Pollack JR, Sikela JM. Gene copy number variation spanning 60 million years of human and primate evolution. Genome Res. 2007; 17 :1266–1277. [ PMC free article ] [ PubMed ] [ Google Scholar ]
- Enard W, Przeworski M, Fisher SE, Lai CS, Wiebe V, Kitano T, Monaco AP, Paabo S. Molecular evolution of FOXP2, a gene involved in speech and language. Nature. 2002; 418 :869–872. [ PubMed ] [ Google Scholar ]
- Fischer A, Pollack J, Thalmann O, Nickel B, Paabo S. Demographic history and genetic differentiation in apes. Curr Biol. 2006; 16 :1133–1138. [ PubMed ] [ Google Scholar ]
- Fortna A, Kim Y, MacLaren E, Marshall K, Hahn G, Meltesen L, Brenton M, Hink R, Burgers S, Hernandez-Boussard T, Karimpour-Fard A, Glueck D, McGavran L, Berry R, Pollack J, Sikela JM. Lineage-specific gene duplication and loss in human and great ape evolution. PLoS Biol. 2004; 2 :E207. [ PMC free article ] [ PubMed ] [ Google Scholar ]
- Frisse L, Hudson RR, Bartoszewicz A, Wall JD, Donfack J, Di Rienzo A. Gene conversion and different population histories may explain the contrast between polymorphism and linkage disequilibrium levels. Am J Hum Genet. 2001; 69 :831–843. [ PMC free article ] [ PubMed ] [ Google Scholar ]
- Galtier N, Duret L. Adaptation or biased gene conversion? Extending the null hypothesis of molecular evolution. Trends Genet. 2007; 23 :273–277. [ PubMed ] [ Google Scholar ]
- Gilad Y, Oshlack A, Smyth GK, Speed TP, White KP. Expression profiling in primates reveals a rapid evolution of human transcription factors. Nature. 2006; 440 :242–245. [ PubMed ] [ Google Scholar ]
- Goidts V, Armengol L, Schempp W, Conroy J, Nowak N, Muller S, Cooper DN, Estivill X, Enard W, Szamalek JM, Hameister H, Kehrer-Sawatzki H. Identification of large-scale human-specific copy number differences by inter-species array comparative genomic hybridization. Hum Genet. 2006; 119 :185–198. [ PubMed ] [ Google Scholar ]
- Goodman M. Epilogue: A personal account of the origins of a new paradigm. Mol Phylogenet Evol. 1996; 5 :269–285. [ PubMed ] [ Google Scholar ]
- Goossens B, Chikhi L, Ancrenaz M, Lackman-Ancrenaz I, Andau P, Bruford MW. Genetic signature of anthropogenic population collapse in orang-utans. PLoS Biol. 2006; 4 e25. [ PMC free article ] [ PubMed ] [ Google Scholar ]
- Groves CP. Order Primates. In: DE Wilson, DM Reeder., editors. Mammal Species of the World. Baltimore: Johns Hopkins University Press. p. 111–184; 2006. [ Google Scholar ]
- Gutenkunst RN, Hernandez RD, Williamson SH, Bustamante CD. Inferring the joint demographic history of multiple populations from multidimensional SNP frequency data. PLoS Genet. 2009; 5 e1000695. [ PMC free article ] [ PubMed ] [ Google Scholar ]
- Hammer MF, Mendez FL, Cox MP, Woerner AE, Wall JD. Sex-biased evolutionary forces shape genomic patterns of human diversity. PLoS Genet. 2008; 4 e1000202. [ PMC free article ] [ PubMed ] [ Google Scholar ]
- Hammer MF, Woerner AE, Mendez FL, Watkins JC, Cox MP, Wall JD. The ratio of human X chromosome to autosome diversity is positively correlated with genetic distance from genes. Nat Genet. 2010; 42 :830–831. [ PubMed ] [ Google Scholar ]
- Harcourt AH, Harvey PH, Larson SG, Short RV. Testis weight, body weight and breeding system in primates. Nature. 1981; 293 :55–57. [ PubMed ] [ Google Scholar ]
- Harding RM, Fullerton SM, Griffiths RC, Clegg JB. A gene tree for beta-globin sequences from Melanesia. J Mol Evol 44 Suppl. 1997; 1 pS133-138. [ PubMed ] [ Google Scholar ]
- Harvati K, Stringer C, Grun R, Aubert M, Allsworth-Jones P, Folorunso CA. PLoS One. Vol. 6. Nigeria: Morphology and chronology; 2011. The Later Stone Age calvaria from Iwo Eleru. e24024. [ PMC free article ] [ PubMed ] [ Google Scholar ]
- Hedrick PW. Population genetics of malaria resistance in humans. Heredity (Edinb) 2011; 107 :283–304. [ PMC free article ] [ PubMed ] [ Google Scholar ]
- Hu HY, He L, Fominykh K, Yan Z, Guo S, Zhang X, Taylor MS, Tang L, Li J, Liu J, Wang W, Yu H, Khaitovich P. Evolution of the human-specific microRNA miR-941. Nat Commun. 2012; 3 :1145. [ PMC free article ] [ PubMed ] [ Google Scholar ]
- Huet T, Cheynier R, Meyerhans A, Roelants G, Wain-Hobson S. Genetic organization of a chimpanzee lentivirus related to HIV-1. Nature. 1990; 345 :356–359. [ PubMed ] [ Google Scholar ]
- Huxley TH. Evidence as to Man's Place in Nature. London England: Williams and Norgate; 1863. [ Google Scholar ]
- IUCN. International Union for Conservation of Nature Red List. 2012 [ Google Scholar ]
- Jolly CJ. A proper study for mankind: Analogies from the Papionin monkeys and their implications for human evolution. Am J Phys Anthropol Suppl. 2001; 33 :177–204. [ PubMed ] [ Google Scholar ]
- Junker J, Blake S, Boesch C, Campbell G, du Toit L, Duvall C, Ekobo A, Etoga G, Galat-Luong A, Gamys J, Ganas-Swaray J, Gatti S, Ghiurghi A, Granier N, Hart J, Head J, Herbinger I, Hicks TC, Huijbregts B, Imong IS, Kuempel N, Lahm S, Lindsell J, Maisels F, McLennan M, Martinez L, Morgan B, Morgan D, Mulindahabi F, Mundry R, N'Goran KP, Normand E, Ntongho A, Okon DT, Petre CA, Plumptre A, Rainey H, Regnaut S, Sanz C, Stokes E, Tondossama A, Tranquilli S, Sunderland-Groves J, Walsh P, Warren Y, Williamson EA, Kuehl HS. Recent decline in suitable environmental conditions for African great apes. Diversity and Distributions. 2012; 18 :1077–1091. [ Google Scholar ]
- Kaessmann H, Wiebe V, Weiss G, Paabo S. Great ape DNA sequences reveal a reduced diversity and an expansion in humans. Nat Genet. 2001; 27 :155–156. [ PubMed ] [ Google Scholar ]
- Katzman S, Kern AD, Pollard KS, Salama SR, Haussler D. GC-biased evolution near human accelerated regions. PLoS Genet. 2010; 6 e1000960. [ PMC free article ] [ PubMed ] [ Google Scholar ]
- Keele BF, Van Heuverswyn F, Li Y, Bailes E, Takehisa J, Santiago ML, Bibollet-Ruche F, Chen Y, Wain LV, Liegeois F, Loul S, Ngole EM, Bienvenue Y, Delaporte E, Brookfield JF, Sharp PM, Shaw GM, Peeters M, Hahn BH. Chimpanzee reservoirs of pandemic and nonpandemic HIV-1. Science. 2006; 313 :523–526. [ PMC free article ] [ PubMed ] [ Google Scholar ]
- Keinan A, Mullikin JC, Patterson N, Reich D. Accelerated genetic drift on chromosome X during the human dispersal out of Africa. Nat Genet. 2009; 41 :66–70. [ PMC free article ] [ PubMed ] [ Google Scholar ]
- Kim HL, Igawa T, Kawashima A, Satta Y, Takahata N. Divergence, demography and gene loss along the human lineage. Philos Trans R Soc Lond B Biol Sci. 2010; 365 :2451–2457. [ PMC free article ] [ PubMed ] [ Google Scholar ]
- Kimura M. Cambridge. United Kingdom: Cambridge University Press; 1983. The Neutral Theory of Molecular Evolution. [ Google Scholar ]
- King MC, Wilson AC. Evolution at two levels in humans and chimpanzees. Science. 1975; 188 :107–116. [ PubMed ] [ Google Scholar ]
- Klein J, Satta Y, Takahata N, O'hUigin C. Trans-specific Mhc polymorphism and the origin of species in primates. J Med Primatol. 1993; 22 :57–64. [ PubMed ] [ Google Scholar ]
- Knowles DG, McLysaght A. Recent de novo origin of human protein-coding genes. Genome Res. 2009; 19 :1752–1759. [ PMC free article ] [ PubMed ] [ Google Scholar ]
- Krause J, Lalueza-Fox C, Orlando L, Enard W, Green RE, Burbano HA, Hublin JJ, Hanni C, Fortea J, de la Rasilla M, Bertranpetit J, Rosas A, Paabo S. The derived FOXP2 variant of modern humans was shared with Neandertals. Curr Biol. 2007; 17 :1908–1912. [ PubMed ] [ Google Scholar ]
- Leffler EM, Gao Z, Pfeifer S, Segurel L, Auton A, Venn O, Bowden R, Bontrop R, Wall JD, Sella G, Donnelly P, McVean G, Przeworski M. Multiple instances of ancient balancing selection shared between humans and chimpanzees. Science. 2013; 339 :1578–1582. [ PMC free article ] [ PubMed ] [ Google Scholar ]
- Lemos B, Meiklejohn CD, Caceres M, Hartl DL. Rates of divergence in gene expression profiles of primates, mice, and flies: Stabilizing selection and variability among functional categories. Evolution. 2005; 59 :126–137. [ PubMed ] [ Google Scholar ]
- Li WH, Sadler LA. Low nucleotide diversity in man. Genetics. 1991; 129 :513–523. [ PMC free article ] [ PubMed ] [ Google Scholar ]
- Linardopoulou EV, Williams EM, Fan Y, Friedman C, Young JM, Trask BJ. Human subtelomeres are hot spots of interchromosomal recombination and segmental duplication. Nature. 2005; 437 :94–100. [ PMC free article ] [ PubMed ] [ Google Scholar ]
- Lindblad-Toh K, Garber M, Zuk O Lin MF, Parker BJ, Washietl S, Kheradpour P, Ernst J, Jordan G, Mauceli E, Ward LD, Lowe CB, Holloway AK, Clamp M, Gnerre S, Alfoldi J, Beal K, Chang J, Clawson H, Cuff J, Di Palma F, Fitzgerald S, Flicek P, Guttman M, Hubisz MJ, Jaffe DB, Jungreis I, Kent WJ, Kostka D, Lara M, Martins AL, Massingham T, Moltke I, Raney BJ, Rasmussen MD, Robinson J, Stark A, Vilella AJ, Wen J, Xie X, Zody MC, Broad Institute Sequencing Platform and Whole Genome Assembly Team, Baldwin J, Bloom T, Chin CW, Heiman D, Nicol R, Nusbaum C, Young S, Wilkinson J, Worley KC, Kovar CL, Muzny DM, Gibbs RA, Baylor College of Medicine Human Genome Sequencing Center Sequencing Team, Cree A, Dihn HH, Fowler G, Jangiani S, Joshi V, Lee S, Lewis LR, Nazareth LV, Okwuonu G, Santibanez J, Warren WC, Mardis ER, Weinstock GM, Wilson RK, Genome Institute at Washington University, Delehaunty K, Dooling D, Fronik C, Fulton L, Fulton B, Graves T, Minx P, Sodergren E, Birney E, Margulies EH, Herrero J, Green ED, Haussler D, Siepel A, Goldman N, Pollard KS, Pedersen JS, Lander ES, Keller M. A high-resolution map of human evolutionary constraint using 29 mammals. Nature. 2011; 478 :476–482. [ PMC free article ] [ PubMed ] [ Google Scholar ]
- Locke DP, Hillier LW, Warren WC, Worley KC, Nazareth LV, Muzny DM, Yang SP, Wang Z, Chinwalla AT, Minx P, Mitreva M, Cook L, Delehaunty KD, Fronick C, Schmidt H, Fulton LA, Fulton RS, Nelson JO, Magrini V, Pohl C, Graves TA, Markovic C, Cree A, Dinh HH, Hume J, Kovar CL, Fowler GR, Lunter G, Meader S, Heger A, Ponting CP, Marques-Bonet T, Alkan C, Chen L, Cheng Z, Kidd JM, Eichler EE, White S, Searle S, Vilella AJ, Chen Y, Flicek P, Ma J, Raney B, Suh B, Burhans R, Herrero J, Haussler D, Faria R, Fernando O, Darré F, Farré D, Gazave E, Oliva M, Navarro A, Roberto R, Capozzi O, Archidiacono N, Della Valle G, Purgato S, Rocchi M, Konkel MK, Walker JA, Ullmer B, Batzer MA, Smit AF, Hubley R, Casola C, Schrider DR, Hahn MW, Quesada V, Puente XS, Ordoñez GR, López-Otín C, Vinar T, Brejova B, Ratan A, Harris RS, Miller W, Kosiol C, Lawson HA, Taliwal V, Martins AL, Siepel A, Roychoudhury A, Ma X, Degenhardt J, Bustamante CD, Gutenkunst RN, Mailund T, Dutheil JY, Hobolth A, Schierup MH, Ryder OA, Yoshinaga Y, de Jong PJ, Weinstock GM, Rogers J, Mardis ER, Gibbs RA, Wilson RK. Comparative and demographic analysis of orang-utan genomes. Nature. 2011; 469 :529–533. [ PMC free article ] [ PubMed ] [ Google Scholar ]
- Maricic T, Gunther V, Georgiev O, Gehre S, Curlin M, Schreiweis C, Naumann R, Burbano HA, Meyer M, Lalueza-Fox C, de la Rasilla M, Rosas A, Gajovic S, Kelso J, Enard W, Schaffner W, Pääbo S. A Recent Evolutionary Change Affects a Regulatory Element in the Human FOXP2 Gene. Mol Biol Evol. 2013; 4 :844–852. [ PubMed ] [ Google Scholar ]
- McDonald JH, Kreitman M. Adaptive protein evolution at the Adh locus in Drosophila. Nature. 1991; 351 :652–654. [ PubMed ] [ Google Scholar ]
- McQuillan R, Leutenegger AL, Abdel-Rahman R, Franklin CS, Pericic M, Barac-Lauc L, Smolej-Narancic N, Janicijevic B, Polasek O, Tenesa A, Macleod AK, Farrington SM, Rudan P, Hayward C, Vitart V, Rudan I, Wild SH, Dunlop MG, Wright AF, Campbell H, Wilson JF. Runs of homozygosity in European populations. Am J Hum Genet. 2008; 83 :359–372. [ PMC free article ] [ PubMed ] [ Google Scholar ]
- Morwood MJ, Brown P, Jatmiko, Sutikna T, Saptomo EW, Westaway KE, Due RA, Roberts RG, Maeda T, Wasisto S, Djubiantono T. Further evidence for small-bodied hominins from the Late Pleistocene of Flores, Indonesia. Nature. 2005; 437 :1012–1017. [ PubMed ] [ Google Scholar ]
- Myers S, Bottolo L, Freeman C, McVean G, Donnelly P. A fine-scale map of recombination rates and hotspots across the human genome. Science. 2005; 310 :321–324. [ PubMed ] [ Google Scholar ]
- Myers S, Bowden R, Tumian A, Bontrop RE, Freeman C, MacFie TS, McVean G, Donnelly P. Drive against hotspot motifs in primates implicates the PRDM9 gene in meiotic recombination. Science. 2010; 327 :876–879. [ PMC free article ] [ PubMed ] [ Google Scholar ]
- Nater A, Arora N, Greminger MP, van Schaik CP, Singleton I, Wich SA, Fredriksson G, Perwitasari-Farajallah D, Pamungkas J, Krutzen M. Marked population structure and recent migration in the critically endangered Sumatran orangutan (Pongo abelii) J Hered. 2013; 104 :2–13. [ PubMed ] [ Google Scholar ]
- Nelson MR, Wegmann D, Ehm MG, Kessner D, St Jean P, Verzilli C, Shen J, Tang Z, Bacanu SA, Fraser D, Warren L, Aponte J, Zawistowski M, Liu X, Zhang H, Zhang Y, Li J, Li Y, Li L, Woollard P, Topp S, Hall MD, Nangle K, Wang J, Abecasis G, Cardon LR, Zöllner S, Whittaker JC, Chissoe SL, Novembre J, Mooser V. An abundance of rare functional variants in 202 drug target genes sequenced in 14,002 people. Science. 2012; 337 :100–104. [ PMC free article ] [ PubMed ] [ Google Scholar ]
- Nielsen R, Bustamante C, Clark AG, Glanowski S, Sackton TB, Hubisz MJ, Fledel-Alon A, Tanenbaum DM, Civello D, White TJ, J Sninsky J, Adams MD, Cargill M. A scan for positively selected genes in the genomes of humans and chimpanzees. PLoS Biol. 2005; 3 e170. [ PMC free article ] [ PubMed ] [ Google Scholar ]
- Nowick K, Gernat T, Almaas E, Stubbs L. Differences in human and chimpanzee gene expression patterns define an evolving network of transcription factors in brain. Proc Natl Acad Sci U S A. 2009; 106 :22358–22363. [ PMC free article ] [ PubMed ] [ Google Scholar ]
- O'hUigin C, Satta Y, Takahata N, Klein J. Contribution of homoplasy and of ancestral polymorphism to the evolution of genes in anthropoid primates. Mol Biol Evol. 2002; 19 :1501–1513. [ PubMed ] [ Google Scholar ]
- Oliver PL, Goodstadt L, Bayes JJ, Birtle Z, Roach KC, Phadnis N, Beatson SA, Lunter G, Malik HS, Ponting CP. Accelerated evolution of the Prdm9 speciation gene across diverse metazoan taxa. PLoS Genet. 2009; 5 e1000753. [ PMC free article ] [ PubMed ] [ Google Scholar ]
- Olson MV. When less is more: Gene loss as an engine of evolutionary change. Am J Hum Genet. 1999; 64 :18–23. [ PMC free article ] [ PubMed ] [ Google Scholar ]
- Pluzhnikov A, Di Rienzo A, Hudson RR. Inferences about human demography based on multilocus analyses of noncoding sequences. Genetics. 2002; 161 :1209–1218. [ PMC free article ] [ PubMed ] [ Google Scholar ]
- Pollard KS, Salama SR, King B, Kern AD, Dreszer T, Katzman S, Siepel A, Pedersen JS, Bejerano G, Baertsch R, Rosenbloom KR, Kent J, Haussler D. Forces shaping the fastest evolving regions in the human genome. PLoS Genet. 2006; 2 e168. [ PMC free article ] [ PubMed ] [ Google Scholar ]
- Pollard KS, Salama SR, Lambert N, Lambot MA, Coppens S, Pedersen JS, Katzman S, King B, Onodera C, Siepel A, Kern AD, Dehay C, Igel H, Ares M, Jr, Vanderhaeghen P, Haussler D. An RNA gene expressed during cortical development evolved rapidly in humans. Nature. 2006; 443 :167–172. [ PubMed ] [ Google Scholar ]
- Prado-Martinez J, Sudmant PH, Kidd JM, Li H, Kelley JL, Lorente-Galdos B, Veeramah KR, Woerner AE, O'Connor TD, Santpere G, Cagan A, Theunert C, Casals F, Laayouni H, Munch K, Hobolth A, Halager AE, Malig M, Hernandez-Rodriguez J, Hernando-Herraez I, Prüfer K, Pybus M, Johnstone L, Lachmann M, Alkan C, Twigg D, Petit N, Baker C, Hormozdiari F, Fernandez-Callejo M, Dabad M, Wilson ML, Stevison L, Camprubí C, Carvalho T, Ruiz-Herrera A, Vives L, Mele M, Abello T, Kondova I, Bontrop RE, Pusey A, Lankester F, Kiyang JA, Bergl RA, Lonsdorf E, Myers S, Ventura M, Gagneux P, Comas D, Siegismund H, Blanc J, Agueda-Calpena L, Gut M, Fulton L, Tishkoff SA, Mullikin JC, Wilson RK, Gut IG, Gonder MK, Ryder OA, Hahn BH, Navarro A, Akey JM, Bertranpetit J, Reich D, Mailund T, Schierup MH, Hvilsom C, Andrés AM, Wall JD, Bustamante CD, Hammer MF, Eichler EE, Marques-Bonet T. Great ape genetic diversity and population history. Nature. 2013; 449 :471–475. [ PMC free article ] [ PubMed ] [ Google Scholar ]
- Prufer K, Munch K, Hellmann I, Akagi K, Miller JR, Walenz B, Koren S, Sutton G, Kodira C, Winer R, Knight JR, Mullikin JC, Meader SJ, Ponting CP, Lunter G, Higashino S, Hobolth A, Dutheil J, Karakoç E, Alkan C, Sajjadian S, Catacchio CR, Ventura M, Marques-Bonet T, Eichler EE, André C, Atencia R, Mugisha L, Junhold J, Patterson N, Siebauer M, Good JM, Fischer A, Ptak SE, Lachmann M, Symer DE, Mailund T, Schierup MH, Andrés AM, Kelso J, Pääbo S. The bonobo genome compared with the chimpanzee and human genomes. Nature. 2012; 486 :527–531. [ PMC free article ] [ PubMed ] [ Google Scholar ]
- Ptak SE, Enard W, Wiebe V, Hellmann I, Krause J, Lachmann M, Paabo S. Linkage disequilibrium extends across putative selected sites in FOXP2. Mol Biol Evol. 2009; 26 :2181–2184. [ PubMed ] [ Google Scholar ]
- Ptak SE, Roeder AE, Stephens M, Gilad Y, Paabo S, Przeworski M. Absence of the TAP2 human recombination hotspot in chimpanzees. PLoS Biol. 2004; 2 e155. [ PMC free article ] [ PubMed ] [ Google Scholar ]
- Reich D, Green RE, Kircher M, Krause J, Patterson N, Durand EY, Viola B, Briggs AW, Stenzel U, Johnson PL, Maricic T, Good JM, Marques-Bonet T, Alkan C, Fu Q, Mallick S, Li H, Meyer M, Eichler EE, Stoneking M, Richards M, Talamo S, Shunkov MV, Derevianko AP, Hublin JJ, Kelso J, Slatkin M, Pääbo S. Genetic history of an archaic hominin group from Denisova Cave in Siberia. Nature. 2010; 468 :1053–1060. [ PMC free article ] [ PubMed ] [ Google Scholar ]
- Rhesus Macaque Genome Sequencing and Analysis Consortium. Evolutionary and biomedical insights from the rhesus macaque genome. Science. 2007; 316 :222–234. [ PubMed ] [ Google Scholar ]
- Rifkin SA, Houle D, Kim J, White KP. A mutation accumulation assay reveals a broad capacity for rapid evolution of gene expression. Nature. 2005; 438 :220–223. [ PubMed ] [ Google Scholar ]
- Romero IG, Ruvinsky I, Gilad Y. Comparative studies of gene expression and the evolution of gene regulation. Nat Rev Genet. 2012; 13 :505–516. [ PMC free article ] [ PubMed ] [ Google Scholar ]
- Satta Y, Klein J, Takahata N. DNA archives and our nearest relative: The trichotomy problem revisited. Mol Phylogenet Evol. 2000; 14 :259–275. [ PubMed ] [ Google Scholar ]
- Scally A, Dutheil JY, Hillier LW, Jordan GE, Goodhead I, Herrero J, Hobolth A, Lappalainen T, Mailund T, Marques-Bonet T, McCarthy S, Montgomery SH, Schwalie PC, Tang YA, Ward MC, Xue Y, Yngvadottir B, Alkan C, Andersen LN, Ayub Q, Ball EV, Beal K, Bradley BJ, Chen Y, Clee CM, Fitzgerald S, Graves TA, Gu Y, Heath P, Heger A, Karakoc E, Kolb-Kokocinski A, Laird GK, Lunter G, Meader S, Mort M, Mullikin JC, Munch K, O'Connor TD, Phillips AD, Prado-Martinez J, Rogers AS, Sajjadian S, Schmidt D, Shaw K, Simpson JT, Stenson PD, Turner DJ, Vigilant L, Vilella AJ, Whitener W, Zhu B, Cooper DN, de Jong P, Dermitzakis ET, Eichler EE, Flicek P, Goldman N, Mundy NI, Ning Z, Odom DT, Ponting CP, Quail MA, Ryder OA, Searle SM, Warren WC, Wilson RK, Schierup MH, Rogers J, Tyler-Smith C, Durbin R. Insights into hominid evolution from the gorilla genome sequence. Nature. 2012; 483 :169–175. [ PMC free article ] [ PubMed ] [ Google Scholar ]
- Segurel L, Thompson EE, Flutre T, Lovstad J, Venkat A, Margulis SW, Moyse J, Ross S, Gamble K, Sella G, Ober C, Przeworski M. The ABO blood group is a trans-species polymorphism in primates. Proc Natl Acad Sci U S A. 2012; 109 :18493–18498. [ PMC free article ] [ PubMed ] [ Google Scholar ]
- Sharp PM, Hahn BH. Origins of HIV and the AIDS pandemic. Cold Spring Harb Perspect Med. 2011; 1 a006841. [ PMC free article ] [ PubMed ] [ Google Scholar ]
- Sibley CG, Ahlquist JE. DNA hybridization evidence of hominoid phylogeny: Results from an expanded data set. J Mol Evol. 1987; 26 :99–121. [ PubMed ] [ Google Scholar ]
- Siepel A, Pollard KS, Haussler D. New methods for detecting lineage-specific selection. Research in Computational Molecular Biology, Proceedings. 2006; 3909 :190–205. [ Google Scholar ]
- Somel M, Franz H, Yan Z, Lorenc A, Guo S, Giger T, Kelso J, Nickel B, Dannemann M, Bahn S, Webster MJ, Weickert CS, Lachmann M, Pääbo S, Khaitovich P. Transcriptional neoteny in the human brain. Proc Natl Acad Sci U S A. 2009; 106 :5743–5748. [ PMC free article ] [ PubMed ] [ Google Scholar ]
- Stahl EA, Dwyer G, Mauricio R, Kreitman M, Bergelson J. Dynamics of disease resistance polymorphism at the Rpm1 locus of Arabidopsis. Nature. 1999; 400 :667–671. [ PubMed ] [ Google Scholar ]
- Stedman HH, Kozyak BW, Nelson A, Thesier DM, Su LT, Low DW, Bridges CR, Shrager JB, Minugh-Purvis N, Mitchell MA. Myosin gene mutation correlates with anatomical changes in the human lineage. Nature. 2004; 428 :415–418. [ PubMed ] [ Google Scholar ]
- Takahata N. Allelic genealogy and human evolution. Mol Biol Evol. 1993; 10 :2–22. [ PubMed ] [ Google Scholar ]
- Takahata N, Satta Y. Evolution of the primate lineage leading to modern humans: Phylogenetic and demographic inferences from DNA sequences. Proc Natl Acad Sci U S A. 1997; 94 :4811–4815. [ PMC free article ] [ PubMed ] [ Google Scholar ]
- Ventura M, Catacchio CR, Sajjadian S, Vives L, Sudmant PH, Marques-Bonet T, Graves TA, Wilson RK, Eichler EE. The evolution of African great ape subtelomeric heterochromatin and the fusion of human chromosome 2. Genome Res. 2012; 22 :1036–1049. [ PMC free article ] [ PubMed ] [ Google Scholar ]
- Voight BF, Adams AM, Frisse LA, Qian Y, Hudson RR, Di Rienzo A. Interrogating multiple aspects of variation in a full resequencing data set to infer human population size changes. Proc Natl Acad Sci U S A. 2005; 102 :18508–18513. [ PMC free article ] [ PubMed ] [ Google Scholar ]
- Wall JD. Estimating ancestral population sizes and divergence times. Genetics. 2003; 163 :395–404. [ PMC free article ] [ PubMed ] [ Google Scholar ]
- Wall JD, Cox MP, Mendez FL, Woerner A, Severson T, Hammer MF. A novel DNA sequence database for analyzing human demographic history. Genome Res. 2008; 18 :1354–1361. [ PMC free article ] [ PubMed ] [ Google Scholar ]
- Wall JD, Frisse LA, Hudson RR, Di Rienzo A. Comparative linkage-disequilibrium analysis of the beta-globin hotspot in primates. Am J Hum Genet. 2003; 73 :1330–1340. [ PMC free article ] [ PubMed ] [ Google Scholar ]
- Wall JD, Slatkin M. Paleopopulation genetics. Annu Rev Genet. 2012; 46 :635–649. [ PMC free article ] [ PubMed ] [ Google Scholar ]
- Wilson GM, Flibotte S, Missirlis PI, Marra MA, Jones S, Thornton K, Clark AG, Holt RA. Identification by full-coverage array CGH of human DNA copy number increases relative to chimpanzee and gorilla. Genome Res. 2006; 16 :173–181. [ PMC free article ] [ PubMed ] [ Google Scholar ]
- Winckler W, Myers SR, Richter DJ, Onofrio RC, McDonald GJ, Bontrop RE, McVean GA, Gabriel SB, Reich D, Donnelly P, Altshuler D. Comparison of fine-scale recombination rates in humans and chimpanzees. Science. 2005; 308 :107–111. [ PubMed ] [ Google Scholar ]
- Wise CA, Sraml M, Rubinsztein DC, Easteal S. Comparative nuclear and mitochondrial genome diversity in humans and chimpanzees. Mol Biol Evol. 1997; 14 :707–716. [ PubMed ] [ Google Scholar ]
- Wu DD, Irwin DD, Zhang YP. De novo origin of human protein-coding genes. PLoS Genet. 2011; 7 e1002379. [ PMC free article ] [ PubMed ] [ Google Scholar ]
- Xu AG, He L, Li Z, Xu Y, Li M, Fu X, Yan Z, Yuan Y, Menzel C, Li N, Somel M, Hu H, Chen W, Pääbo S, Khaitovich P. Intergenic and repeat transcription in human, chimpanzee and macaque brains measured by RNA-Seq. PLoS Comput Biol. 2010; 6 e1000843. [ PMC free article ] [ PubMed ] [ Google Scholar ]
- Xu X, Arnason U. The mitochondrial DNA molecule of Sumatran orangutan and a molecular proposal for two (Bornean and Sumatran) species of orangutan. J Mol Evol. 1996; 43 :431–437. [ PubMed ] [ Google Scholar ]
- Xue Y, Daly A, Yngvadottir B, Liu M, Coop G, Kim Y, Sabeti P, Chen Y, Stalker J, Huckle E, Burton J, Leonard S, Rogers J, Tyler-Smith C. Spread of an inactive form of Caspase-12 in humans is due to recent positive selection. Am J Hum Genet. 2006; 78 :659–670. [ PMC free article ] [ PubMed ] [ Google Scholar ]
- Yunis JJ, Prakash O. The origin of man: A chromosomal pictorial legacy. Science. 1982; 215 :1525–1530. [ PubMed ] [ Google Scholar ]
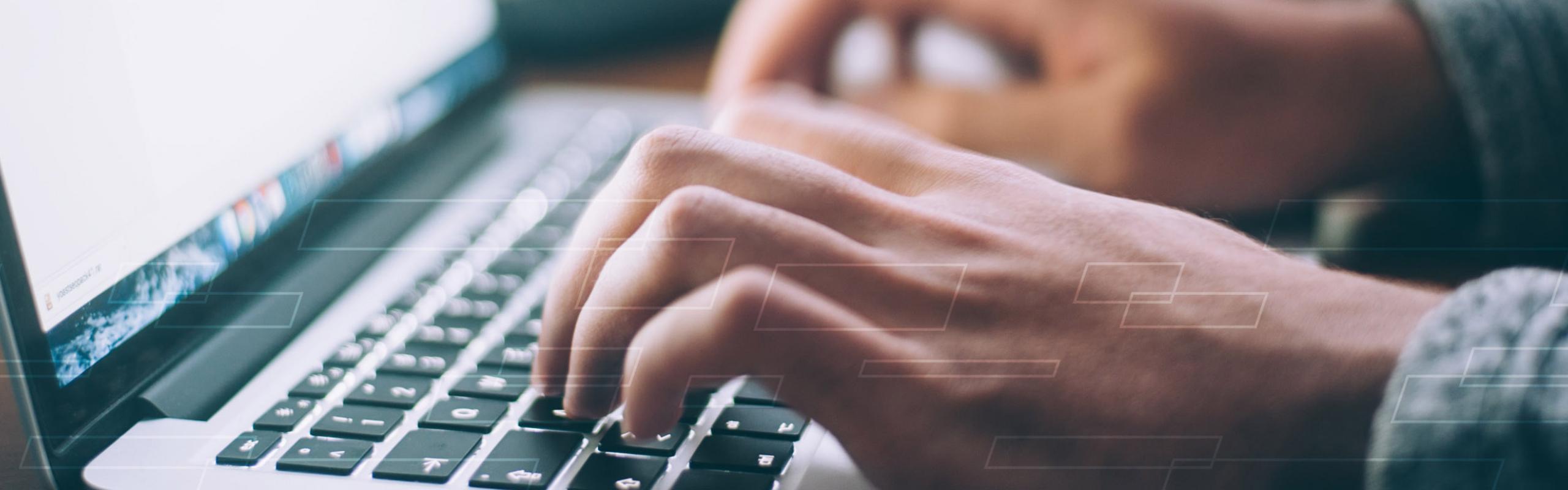
User Preferences
Content preview.
Arcu felis bibendum ut tristique et egestas quis:
- Ut enim ad minim veniam, quis nostrud exercitation ullamco laboris
- Duis aute irure dolor in reprehenderit in voluptate
- Excepteur sint occaecat cupidatat non proident
Keyboard Shortcuts
S.3.3 hypothesis testing examples.
- Example: Right-Tailed Test
- Example: Left-Tailed Test
- Example: Two-Tailed Test
Brinell Hardness Scores
An engineer measured the Brinell hardness of 25 pieces of ductile iron that were subcritically annealed. The resulting data were:
Brinell Hardness of 25 Pieces of Ductile Iron | ||||||||
---|---|---|---|---|---|---|---|---|
170 | 167 | 174 | 179 | 179 | 187 | 179 | 183 | 179 |
156 | 163 | 156 | 187 | 156 | 167 | 156 | 174 | 170 |
183 | 179 | 174 | 179 | 170 | 159 | 187 |
The engineer hypothesized that the mean Brinell hardness of all such ductile iron pieces is greater than 170. Therefore, he was interested in testing the hypotheses:
H 0 : μ = 170 H A : μ > 170
The engineer entered his data into Minitab and requested that the "one-sample t -test" be conducted for the above hypotheses. He obtained the following output:
Descriptive Statistics
N | Mean | StDev | SE Mean | 95% Lower Bound |
---|---|---|---|---|
25 | 172.52 | 10.31 | 2.06 | 168.99 |
$\mu$: mean of Brinelli
Null hypothesis H₀: $\mu$ = 170 Alternative hypothesis H₁: $\mu$ > 170
T-Value | P-Value |
---|---|
1.22 | 0.117 |
The output tells us that the average Brinell hardness of the n = 25 pieces of ductile iron was 172.52 with a standard deviation of 10.31. (The standard error of the mean "SE Mean", calculated by dividing the standard deviation 10.31 by the square root of n = 25, is 2.06). The test statistic t * is 1.22, and the P -value is 0.117.
If the engineer set his significance level α at 0.05 and used the critical value approach to conduct his hypothesis test, he would reject the null hypothesis if his test statistic t * were greater than 1.7109 (determined using statistical software or a t -table):
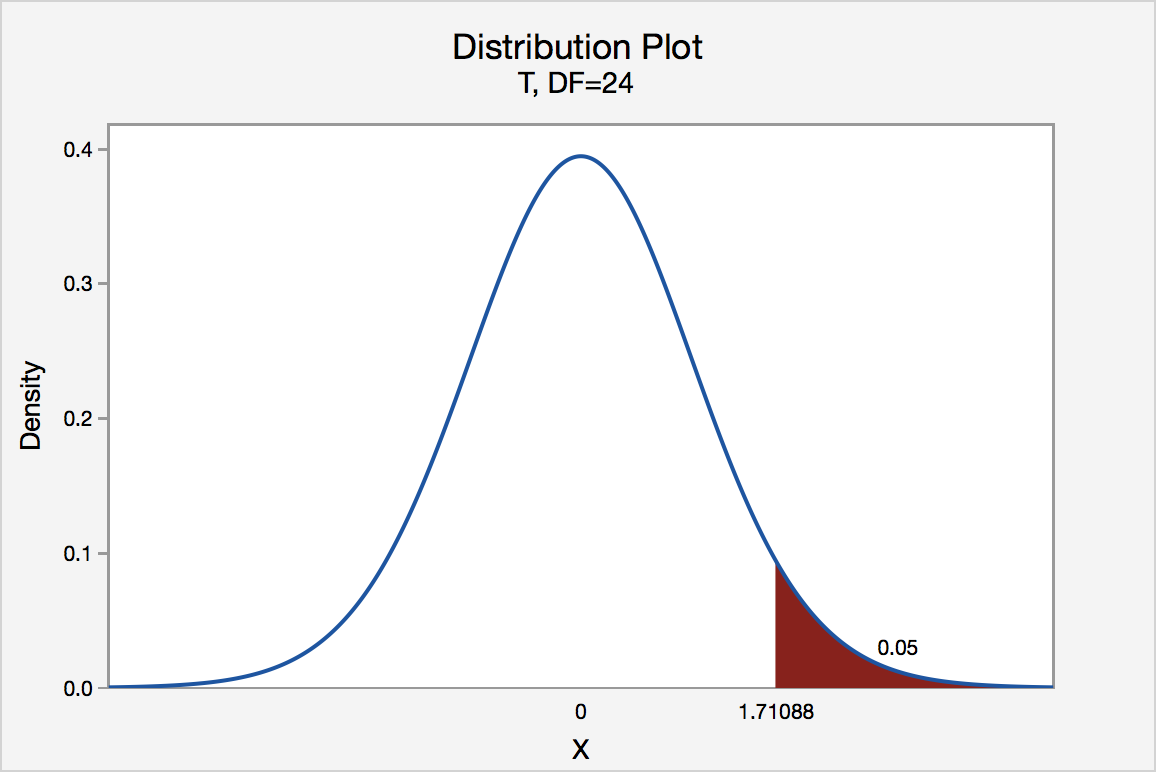
Since the engineer's test statistic, t * = 1.22, is not greater than 1.7109, the engineer fails to reject the null hypothesis. That is, the test statistic does not fall in the "critical region." There is insufficient evidence, at the \(\alpha\) = 0.05 level, to conclude that the mean Brinell hardness of all such ductile iron pieces is greater than 170.
If the engineer used the P -value approach to conduct his hypothesis test, he would determine the area under a t n - 1 = t 24 curve and to the right of the test statistic t * = 1.22:
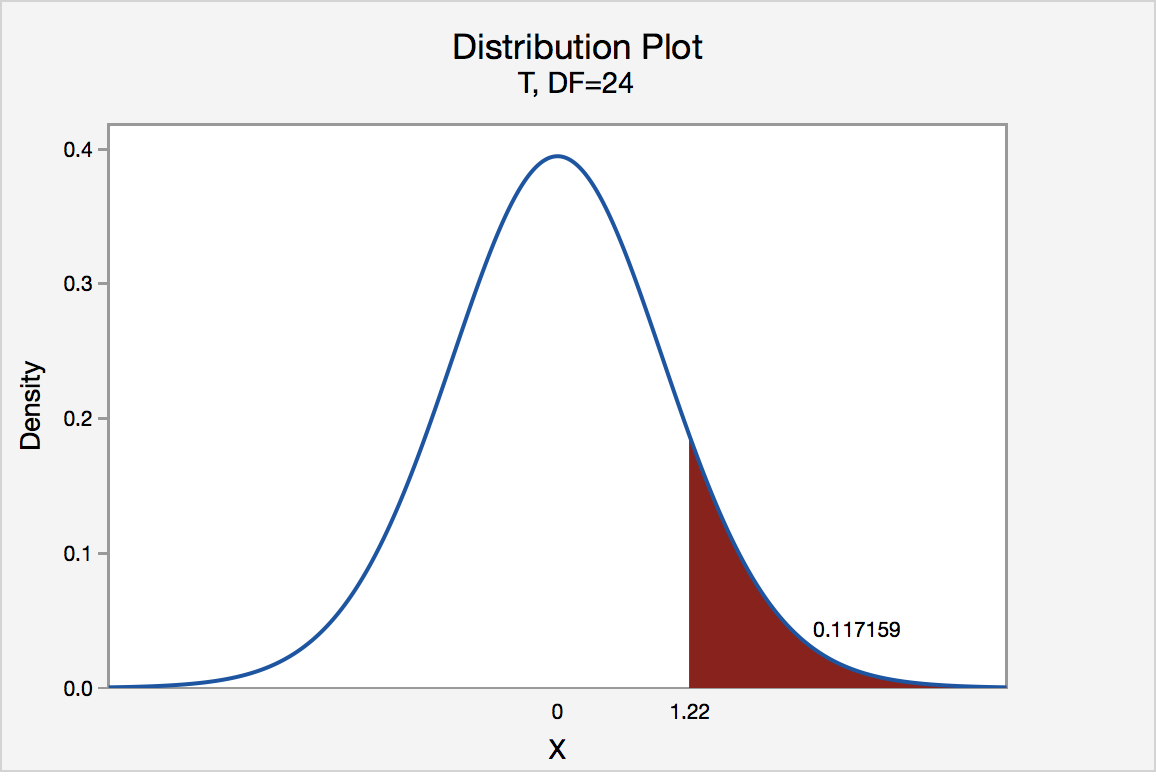
In the output above, Minitab reports that the P -value is 0.117. Since the P -value, 0.117, is greater than \(\alpha\) = 0.05, the engineer fails to reject the null hypothesis. There is insufficient evidence, at the \(\alpha\) = 0.05 level, to conclude that the mean Brinell hardness of all such ductile iron pieces is greater than 170.
Note that the engineer obtains the same scientific conclusion regardless of the approach used. This will always be the case.
Height of Sunflowers
A biologist was interested in determining whether sunflower seedlings treated with an extract from Vinca minor roots resulted in a lower average height of sunflower seedlings than the standard height of 15.7 cm. The biologist treated a random sample of n = 33 seedlings with the extract and subsequently obtained the following heights:
Heights of 33 Sunflower Seedlings | ||||||||
---|---|---|---|---|---|---|---|---|
11.5 | 11.8 | 15.7 | 16.1 | 14.1 | 10.5 | 9.3 | 15.0 | 11.1 |
15.2 | 19.0 | 12.8 | 12.4 | 19.2 | 13.5 | 12.2 | 13.3 | |
16.5 | 13.5 | 14.4 | 16.7 | 10.9 | 13.0 | 10.3 | 15.8 | |
15.1 | 17.1 | 13.3 | 12.4 | 8.5 | 14.3 | 12.9 | 13.5 |
The biologist's hypotheses are:
H 0 : μ = 15.7 H A : μ < 15.7
The biologist entered her data into Minitab and requested that the "one-sample t -test" be conducted for the above hypotheses. She obtained the following output:
N | Mean | StDev | SE Mean | 95% Upper Bound |
---|---|---|---|---|
33 | 13.664 | 2.544 | 0.443 | 14.414 |
$\mu$: mean of Height
Null hypothesis H₀: $\mu$ = 15.7 Alternative hypothesis H₁: $\mu$ < 15.7
T-Value | P-Value |
---|---|
-4.60 | 0.000 |
The output tells us that the average height of the n = 33 sunflower seedlings was 13.664 with a standard deviation of 2.544. (The standard error of the mean "SE Mean", calculated by dividing the standard deviation 13.664 by the square root of n = 33, is 0.443). The test statistic t * is -4.60, and the P -value, 0.000, is to three decimal places.
Minitab Note. Minitab will always report P -values to only 3 decimal places. If Minitab reports the P -value as 0.000, it really means that the P -value is 0.000....something. Throughout this course (and your future research!), when you see that Minitab reports the P -value as 0.000, you should report the P -value as being "< 0.001."
If the biologist set her significance level \(\alpha\) at 0.05 and used the critical value approach to conduct her hypothesis test, she would reject the null hypothesis if her test statistic t * were less than -1.6939 (determined using statistical software or a t -table):s-3-3
Since the biologist's test statistic, t * = -4.60, is less than -1.6939, the biologist rejects the null hypothesis. That is, the test statistic falls in the "critical region." There is sufficient evidence, at the α = 0.05 level, to conclude that the mean height of all such sunflower seedlings is less than 15.7 cm.
If the biologist used the P -value approach to conduct her hypothesis test, she would determine the area under a t n - 1 = t 32 curve and to the left of the test statistic t * = -4.60:
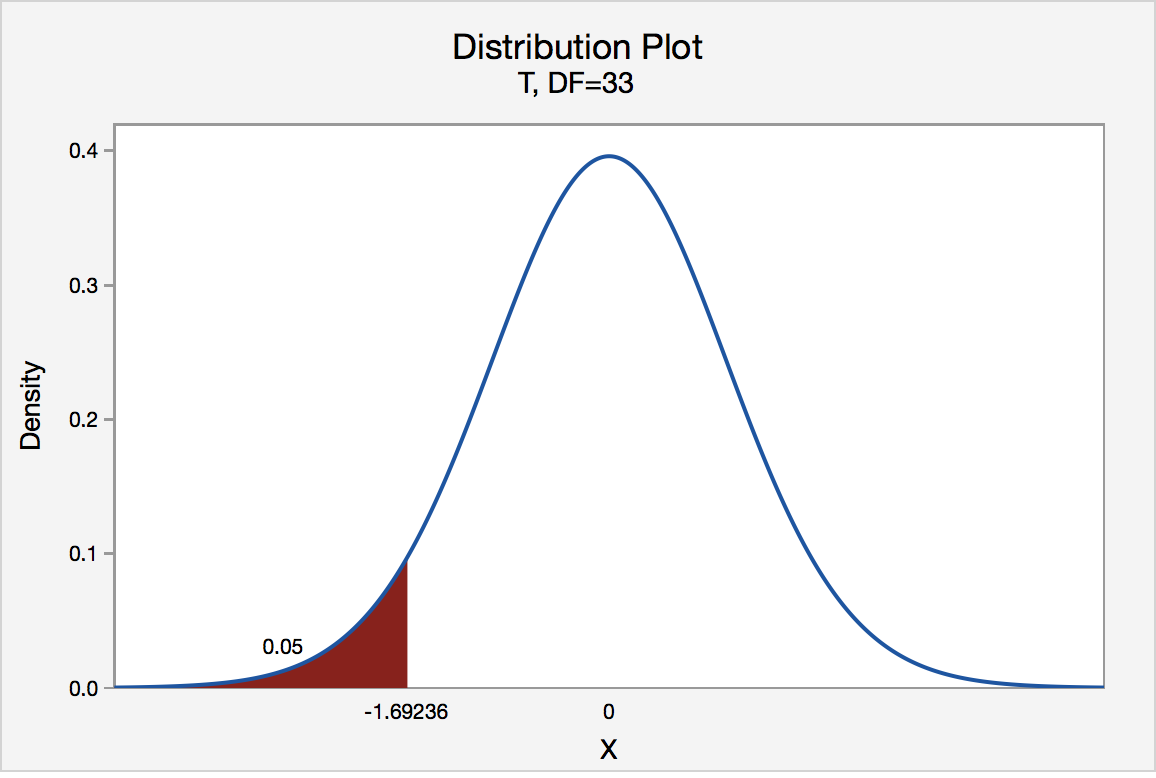
In the output above, Minitab reports that the P -value is 0.000, which we take to mean < 0.001. Since the P -value is less than 0.001, it is clearly less than \(\alpha\) = 0.05, and the biologist rejects the null hypothesis. There is sufficient evidence, at the \(\alpha\) = 0.05 level, to conclude that the mean height of all such sunflower seedlings is less than 15.7 cm.
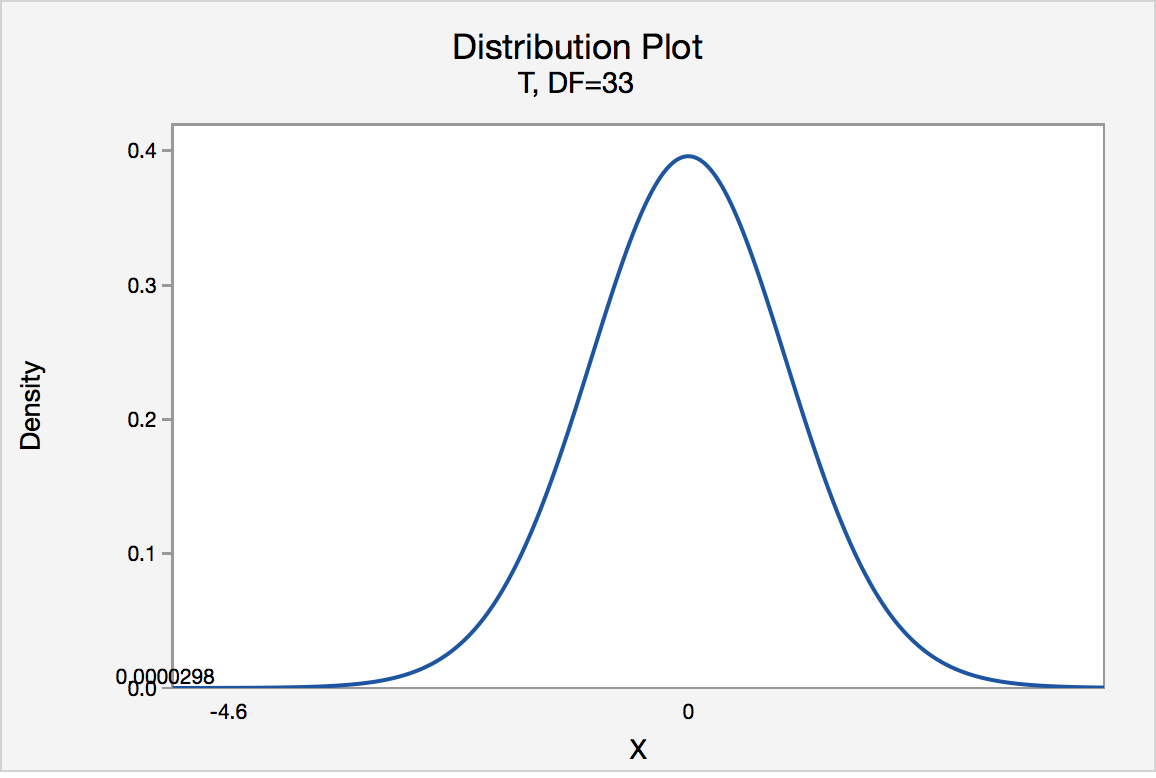
Note again that the biologist obtains the same scientific conclusion regardless of the approach used. This will always be the case.
Gum Thickness
A manufacturer claims that the thickness of the spearmint gum it produces is 7.5 one-hundredths of an inch. A quality control specialist regularly checks this claim. On one production run, he took a random sample of n = 10 pieces of gum and measured their thickness. He obtained:
Thicknesses of 10 Pieces of Gum | ||||
---|---|---|---|---|
7.65 | 7.60 | 7.65 | 7.70 | 7.55 |
7.55 | 7.40 | 7.40 | 7.50 | 7.50 |
The quality control specialist's hypotheses are:
H 0 : μ = 7.5 H A : μ ≠ 7.5
The quality control specialist entered his data into Minitab and requested that the "one-sample t -test" be conducted for the above hypotheses. He obtained the following output:
N | Mean | StDev | SE Mean | 95% CI for $\mu$ |
---|---|---|---|---|
10 | 7.550 | 0.1027 | 0.0325 | (7.4765, 7.6235) |
$\mu$: mean of Thickness
Null hypothesis H₀: $\mu$ = 7.5 Alternative hypothesis H₁: $\mu \ne$ 7.5
T-Value | P-Value |
---|---|
1.54 | 0.158 |
The output tells us that the average thickness of the n = 10 pieces of gums was 7.55 one-hundredths of an inch with a standard deviation of 0.1027. (The standard error of the mean "SE Mean", calculated by dividing the standard deviation 0.1027 by the square root of n = 10, is 0.0325). The test statistic t * is 1.54, and the P -value is 0.158.
If the quality control specialist sets his significance level \(\alpha\) at 0.05 and used the critical value approach to conduct his hypothesis test, he would reject the null hypothesis if his test statistic t * were less than -2.2616 or greater than 2.2616 (determined using statistical software or a t -table):
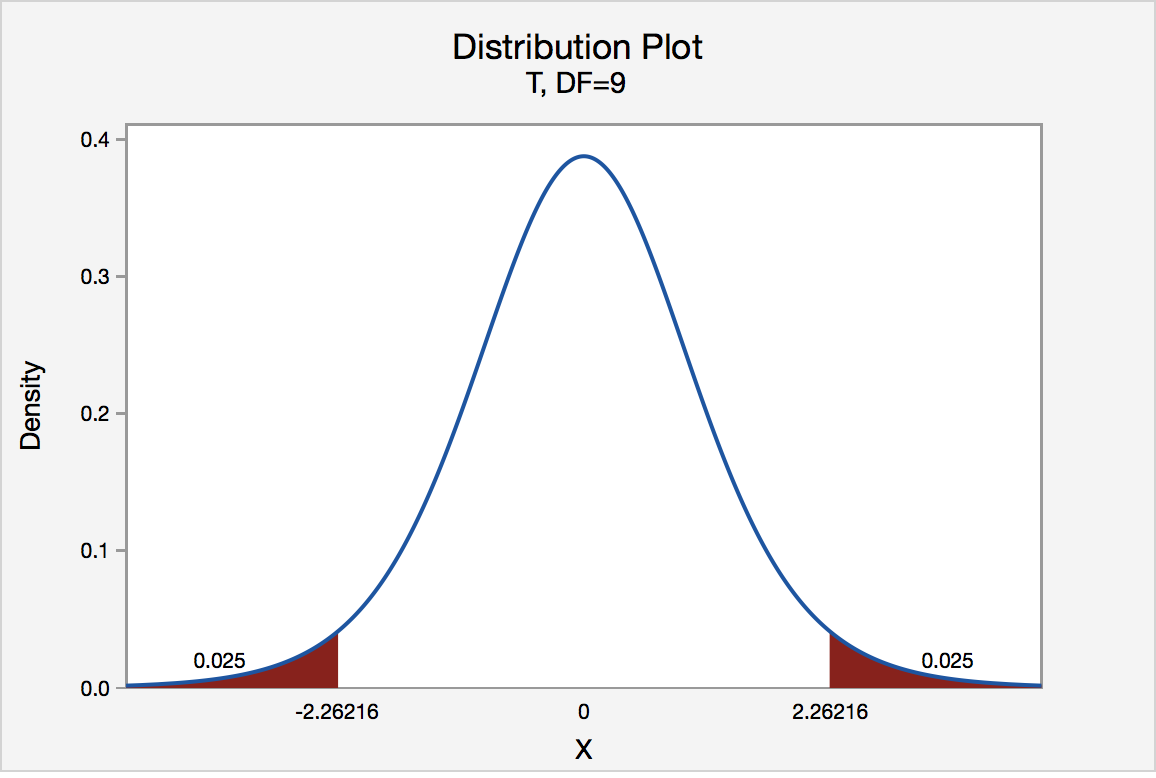
Since the quality control specialist's test statistic, t * = 1.54, is not less than -2.2616 nor greater than 2.2616, the quality control specialist fails to reject the null hypothesis. That is, the test statistic does not fall in the "critical region." There is insufficient evidence, at the \(\alpha\) = 0.05 level, to conclude that the mean thickness of all of the manufacturer's spearmint gum differs from 7.5 one-hundredths of an inch.
If the quality control specialist used the P -value approach to conduct his hypothesis test, he would determine the area under a t n - 1 = t 9 curve, to the right of 1.54 and to the left of -1.54:
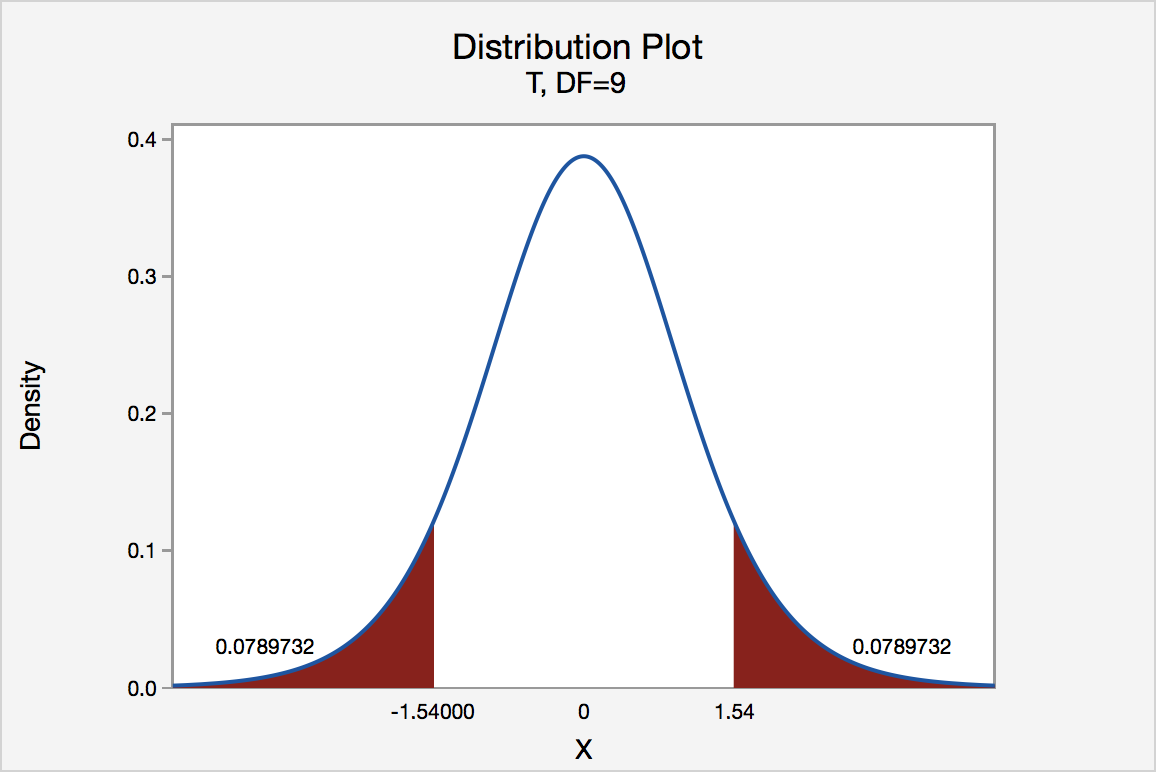
In the output above, Minitab reports that the P -value is 0.158. Since the P -value, 0.158, is greater than \(\alpha\) = 0.05, the quality control specialist fails to reject the null hypothesis. There is insufficient evidence, at the \(\alpha\) = 0.05 level, to conclude that the mean thickness of all pieces of spearmint gum differs from 7.5 one-hundredths of an inch.
Note that the quality control specialist obtains the same scientific conclusion regardless of the approach used. This will always be the case.
In our review of hypothesis tests, we have focused on just one particular hypothesis test, namely that concerning the population mean \(\mu\). The important thing to recognize is that the topics discussed here — the general idea of hypothesis tests, errors in hypothesis testing, the critical value approach, and the P -value approach — generally extend to all of the hypothesis tests you will encounter.

IMAGES
VIDEO
COMMENTS
The example examined in this chapter is the Aquatic Ape Hypothesis, which proposes that important human characteristics were originally adaptations to an ecological niche in or near the water. Unlike many conventional umbrella hypotheses, this one emerged within a separate paradigm that has not yet been reconciled to mainstream paleoanthropology.
Aquatic ape hypothesis
Primate Speciation: A Case Study of African Apes
A New Aquatic Ape Theory
Why are there apes? Evidence for the co‐evolution ...
Primate Cognition | Learn Science at Scitable
Thus, the updated hypothesis of phylogenetic relationships presented here can be used to gain a better understanding of important morphological transitions that took place during hominid evolution, ancestral morphotypes at key nodes, and the biogeography of the clade. Phylogenetic analysis of Middle-Late Miocene apes. Kelsey D. Pugh
In the case of ape tool use, for example, re-representations may allow an individual to generate representations of competing techniques and compare them to solve a problem ... As of now, the empirical data are consistent with what we call the 'Jourdain Hypothesis,' which states that apes have culture, but do not know that they do, in ...
The Aquatic Ape Theory or Aquatic Ape Hypothesis (aka AAT or AAH—this entry will refer to it as the AAT/H) hypothesizes that humans went through an aquatic or semiaquatic stage in our evolution, generally said to have occurred during the transition from the last common ancestor we shared with apes (LCA) to hominids (some, like Marc Verhaegen ...
For example, Tomasello and Carpenter found that three young, human ... The hypothesis that great ape skills of metacognition and social cognition are intimately related is one that should be further explored in great apes and other species. More specifically, the current hypothesis would make the following five predictions, all of which could ...
How closely related are humans to apes and other animals ...
The resulting Jourdain Hypothesis, based on Molière's character, argues that apes express their cultures without knowing that they are cultural beings because of cognitive limitations in their ability to represent knowledge, a determining feature of modern human cultures, allowing representing and modifying the current norms of the group ...
scientists use a null hypothesis. A null hypothesis is a prediction that there is no difference between groups or conditions, or a statement or idea that can be falsified, or proved wrong. The statement "Fish deaths have no relationship to something in the water" is an example of a null hypothesis. Scientific method An objective method to
Here are some research hypothesis examples: If you leave the lights on, then it takes longer for people to fall asleep. If you refrigerate apples, they last longer before going bad. If you keep the curtains closed, then you need less electricity to heat or cool the house (the electric bill is lower). If you leave a bucket of water uncovered ...
15 Hypothesis Examples. A hypothesis is defined as a testable prediction, and is used primarily in scientific experiments as a potential or predicted outcome that scientists attempt to prove or disprove (Atkinson et al., 2021; Tan, 2022). In my types of hypothesis article, I outlined 13 different hypotheses, including the directional hypothesis ...
Hypothesis: Definition, Examples, and Types
Examples of null and alternative hypotheses (video)
Hypothesis Testing | A Step-by-Step Guide with Easy ...
What Are Examples of a Hypothesis?
How to Write a Strong Hypothesis | Steps & Examples
What is a research hypothesis: How to write it, types, and ...
The great ape families are the species most closely related to our own, comprising chimpanzees, bonobos, gorillas, and orangutans. They live exclusively in tropical rainforests in Central Africa and the islands of Southeast Asia. Due to their close evolutionary relationship with humans, great apes share many cognitive, physiological, and ...
S.3.3 Hypothesis Testing Examples | STAT ONLINE